Versatile Polymer Architectures: The Science and Applications of Copolymers
This essay talks about copolymerization and focuses on environmentally friendly ways such as combining CO2 and epoxide. We talk about new technologies, where they’re going, and how they can be used in biomedicine and eco-friendly products.
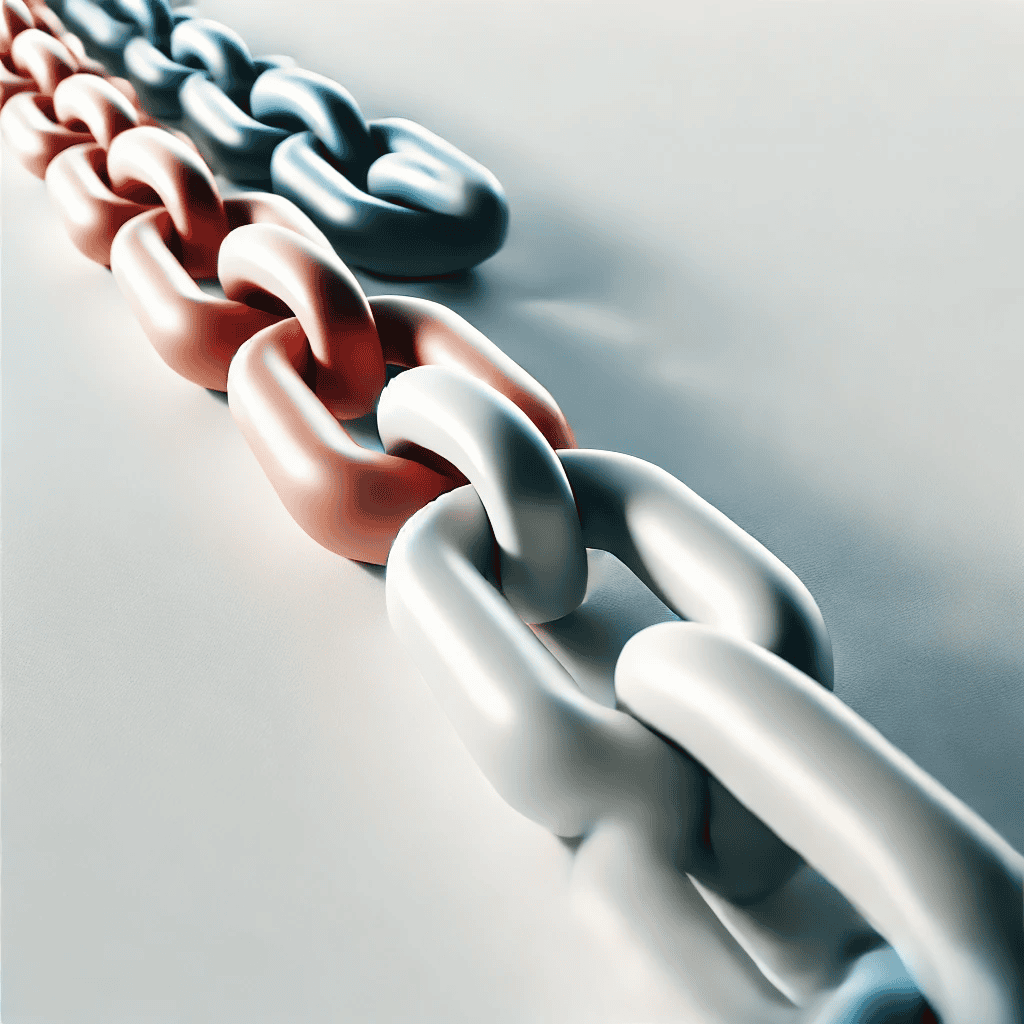
Introduction to Copolymers
Copolymers are long chains made up of two or more different monomeric species. These species can be ordered in different ways, such as in a block, a random, or an alternating pattern. They are important because they can mix the properties of different monomers to make materials with specific properties that can be used in many areas, from medicine to high-tech materials (Park et al., 2018; Ahmad et al., 2010). Because copolymers are so flexible, they can be used to make materials that meet certain performance standards, such as better mechanical strength, thermal stability, and biodegradability. These are all things that are becoming more and more important in the context of sustainable materials (Lin et al., 2021; Lee et al., 2013).
The history of copolymerization, especially with epoxides and carbon dioxide (CO2), has become more popular as a way to make long-lasting polymers. Copolymerization of epoxides with CO2 is not only a way to make polycarbonates, but it also helps the climate by using CO2, which is a greenhouse gas, as a feedstock (Longo et al., 2016; Lee et al., 2015). The copolymerization process is now much more efficient and selective thanks to improvements in catalyst design and reaction conditions (Wang et al., 2023) in this process. The addition of CO2 to polymer chains not only helps lower carbon emissions, but it also gives the materials special qualities that make them useful for packaging, coatings, and healthcare devices (Kai et al., 2016; Jia et al., 2022).
People are interested in copolymers right now, especially those made from renewable resources, because we need long-lasting products that can replace polymers made from petroleum. For example, copolymers made from biobased monomers like itaconate and lactide have shown potential in getting good mechanical properties while still being eco-friendly (Pirman, 2023; Gallagher & Reineke, 2016). People are looking into using these materials for many things, like pressure-sensitive adhesives, drug delivery systems, and recyclable packaging (Vendamme et al., 2014; Loh et al., 2008). Copolymers are being used in new and interesting ways in the biomedical field, like when thermogelling copolymers were created for long-term drug transport and tissue engineering (Loh et al., 2009). Adding bio-derived parts to copolymer mixtures not only makes them last longer, but it also makes it possible to functionalize them in ways that can make them work better in certain situations (Vendamme & Eevers, 2013).
To sum up, copolymers are an important area of study and research in materials science, especially when it comes to sustainability. The progress made in the past few decades in epoxide and CO2 copolymerization has paved the way for new ways to make polymers that are better for the environment. Copolymers will likely be used in more businesses as people become more interested in eco-friendly materials. This will help make the future more eco-friendly.
Technological Advancements in Synthesis
Recent improvements in catalyst design and synthesis methods have changed copolymer synthesis in a big way, making it more efficient and selective. The most important advances in catalyst design have been in making new catalytic systems that allow for more controlled polymerization processes. For example, adding α-diimine palladium catalysts has shown better thermal stability and higher rates of polar monomers incorporation in copolymerization processes, which leads to the creation of high-molecular-weight copolymers (Guo et al., 2015; Guo et al., 2012). The use of dibenzobarrelene-based α-diimine palladium catalysts has also made copolymerizations between carbon monoxide and styrene more productive and easier to control (Xiao et al., 2018). These improvements show that people are moving toward using stronger and more specific catalysts that can work in milder conditions and with more types of monomers.
Copolymerization processes have become more efficient and selective thanks in large part to changes in the ways they are made. Atom Transfer Radical Polymerization (ATRP) and other techniques like it have become popular because they can make copolymers with controlled structures (Matyjaszewski, 2018). ATRP lets different functions be added to the polymer backbone, which makes it possible to make complex materials with specific qualities (Matyjaszewski, 2018). Also, the creation of programmable high-throughput platforms has made it easier to make large amounts of polyester and polycarbonate libraries quickly. This shows that automated synthesis methods could be used to speed up the production of polymers (Lin et al., 2019). It is now much easier to easily look into a huge range of copolymer compositions and architectures thanks to the move toward high-throughput methods.
When old techniques are compared to new ones, it is clear that the new ones give you a lot more control over the structure and function of polymers. Using old ways of polymerization often made materials that were spread out and didn’t let you control the molecular weight and makeup very well. Modern methods, on the other hand, like RAFT (Reversible Addition-Fragmentation Chain Transfer) polymerization and living coordination polymerization, give us better control over the molecular weight distribution and make it possible to precisely synthesize block copolymers (Giaouzi & Pispas, 2019). Using continuous flow polymerization methods together has also become an interesting way to make complex polymer structures while reducing waste and raising reaction efficiency (Reis et al., 2020). These improvements not only make the synthesized materials better, but they also meet the growing need in the polymer business for environmentally friendly and cost-effective ways to make things.
In conclusion, improvements in catalyst design and synthesis methods have changed copolymer synthesis in a big way, making it more efficient, selective, and long-lasting. The change from old-fashioned polymerization methods to newer controlled radical polymerization methods shows how important new ideas are in the field. They make it possible to create next-generation materials with specific qualities for different uses.
Future Directions and Challenges
Researchers are looking into where copolymer synthesis could go in the future because it could be used in many areas, such as biological engineering, packaging, and nanotechnology. The creation of “smart” materials that can react to changes in their surroundings, like temperature or pH, is one of the most exciting areas. For example, amphiphilic block copolymers have a lot of promise in drug delivery systems because they can self-assemble into micelles that can hold hydrophobic drugs and release them slowly (Boadi et al., 2020; Nazemi et al., 2016). Making biodegradable copolymers, like lactide-valerolactone copolymers, is also becoming more popular for use in packaging, where sustainability is becoming more important (Sangroniz et al., 2021). Lignin-based copolymers being added to functional materials is also a big step toward making environmentally friendly options for many uses (Kai et al., 2016).
Even with these improvements, there are still some problems that make it hard to make copolymers. One big problem is that it’s hard to make copolymers while keeping exact control over their structure and molecular weight. Materials made with traditional ways are often polydisperse, which can make them less useful in some situations (Jang et al., 2021). Also, the ability of new synthesis techniques to be used on a large scale is still a worry, since many of these new methods have only been tested in the lab and might not work well in industrial production (Reis et al., 2021). Also, quick and safe ways to make chemicals are needed because the polymer business is getting more and more attention for how it affects the environment (Lin et al., 2019).
The future of copolymer study is expected to be shaped by a number of new ideas and trends. Artificial intelligence and machine learning used in polymer synthesis should make it easier to find and improve new copolymer mixtures (Reis et al., 2021). Researchers could study a wider range of chemicals more quickly and easily with this method because it could cut down on the time and resources needed for experiments. Meanwhile, improvements in continuous flow polymerization methods are expected to make copolymer production more scalable and efficient, making it possible to make complicated structures with little waste (Reis et al., 2021). As self-switchable polymerization systems get better, they could help make new materials with the right properties for different uses, like glue and elastomers (Xia et al., 2021). Overall, the field of copolymer synthesis is about to make big steps forward that will fix current problems and make these materials more useful in more situations.
References:
Ahmad, F., Baloch, M., Jamil, M., & Jeon, Y. (2010). Characterization of polystyrene‐b‐poly(ethylene oxide) diblock copolymer and investigation of its micellization behavior in water. Journal of Applied Polymer Science, 118(3), 1704-1712. https://doi.org/10.1002/app.32165
Gallagher, J. and Reineke, T. (2016). Acrylic triblock copolymers incorporating isosorbide for pressure sensitive adhesives. Acs Sustainable Chemistry & Engineering, 4(6), 3379-3387. https://doi.org/10.1021/acssuschemeng.6b00455
Jia, Y., Sun, Z., Hu, C., & Pang, X. (2022). Switchable polymerization: a practicable strategy to produce biodegradable block copolymers with diverse properties. Chempluschem, 87(9). https://doi.org/10.1002/cplu.202200220
Kai, D., Ren, W., Tian, L., Chee, P., Liu, Y., Ramakrishna, S., … & Loh, X. (2016). Engineering poly(lactide)–lignin nanofibers with antioxidant activity for biomedical application. Acs Sustainable Chemistry & Engineering, 4(10), 5268-5276. https://doi.org/10.1021/acssuschemeng.6b00478
Lee, I., Panthani, T., & Bates, F. (2013). Sustainable poly(lactide-b-butadiene) multiblock copolymers with enhanced mechanical properties. Macromolecules, 46(18), 7387-7398. https://doi.org/10.1021/ma401508b
Lee, S., Lee, K., Kim, Y., & Shin, J. (2015). Preparation and characterization of a renewable pressure-sensitive adhesive system derived from ε-decalactone, l-lactide, epoxidized soybean oil, and rosin ester. Acs Sustainable Chemistry & Engineering, 3(9), 2309-2320. https://doi.org/10.1021/acssuschemeng.5b00580
Lin, Q., Lim, J., Chee, P., Yew, M., Hor, E., & Loh, X. (2021). The thermogel chronicle─from rational design of thermogelling copolymers to advanced thermogel applications. Accounts of Materials Research, 2(10), 881-894. https://doi.org/10.1021/accountsmr.1c00128
Loh, X., Goh, S., & Li, J. (2009). Biodegradable thermogelling poly[(r)-3-hydroxybutyrate]-based block copolymers: micellization, gelation, and cytotoxicity and cell culture studies. The Journal of Physical Chemistry B, 113(35), 11822-11830. https://doi.org/10.1021/jp903984r
Loh, X., Tan, Y., Li, Z., Teo, L., Goh, S., & Li, J. (2008). Biodegradable thermogelling poly(ester urethane)s consisting of poly(lactic acid) – thermodynamics of micellization and hydrolytic degradation. Biomaterials, 29(14), 2164-2172. https://doi.org/10.1016/j.biomaterials.2008.01.016
Longo, J., Sanford, M., & Coates, G. (2016). Ring-opening copolymerization of epoxides and cyclic anhydrides with discrete metal complexes: structure–property relationships. Chemical Reviews, 116(24), 15167-15197. https://doi.org/10.1021/acs.chemrev.6b00553
Park, M., Lee, S., Kim, A., Choi, I., Shin, J., & Kim, Y. (2018). Toughened and hydrophobically modified polyamide 11 copolymers with dimer acids derived from waste vegetable oil. Journal of Applied Polymer Science, 136(10). https://doi.org/10.1002/app.47174
Pirman, T. (2023). Free-radical homopolymerization kinetics of biobased dibutyl itaconate. Acs Applied Polymer Materials, 5(11), 9213-9224. https://doi.org/10.1021/acsapm.3c01708
Vendamme, R. and Eevers, W. (2013). Sweet solution for sticky problems: chemoreological design of self-adhesive gel materials derived from lipid biofeedstocks and adhesion tailoring via incorporation of isosorbide. Macromolecules, 46(9), 3395-3405. https://doi.org/10.1021/ma400203v
Vendamme, R., Schüwer, N., & Eevers, W. (2014). Recent synthetic approaches and emerging bio‐inspired strategies for the development of sustainable pressure‐sensitive adhesives derived from renewable building blocks. Journal of Applied Polymer Science, 131(17). https://doi.org/10.1002/app.40669
Wang, X., Huo, Z., Xie, X., Shanaiah, N., & Tong, R. (2023). Recent advances in sequence‐controlled ring‐opening copolymerizations of monomer mixtures. Chemistry – An Asian Journal, 18(4). https://doi.org/10.1002/asia.202201147
Giaouzi, D. and Pispas, S. (2019). Synthesis and self‐assembly of thermoresponsive poly(n‐isopropylacrylamide)‐b‐poly(oligo ethylene glycol methyl ether acrylate) double hydrophilic block copolymers. Journal of Polymer Science Part a Polymer Chemistry, 57(13), 1467-1477. https://doi.org/10.1002/pola.29411
Guo, L., Dai, S., Sui, X., & Chen, C. (2015). Palladium and nickel catalyzed chain walking olefin polymerization and copolymerization. Acs Catalysis, 6(1), 428-441. https://doi.org/10.1021/acscatal.5b02426
Guo, L., Gao, H., Guan, Q., Hu, H., Deng, J., Liu, J., … & Wu, Q. (2012). Substituent effects of the backbone in α-diimine palladium catalysts on homo- and copolymerization of ethylene with methyl acrylate. Organometallics, 31(17), 6054-6062. https://doi.org/10.1021/om300380b
Lin, B., Hedrick, J., Park, N., & Waymouth, R. (2019). Programmable high-throughput platform for the rapid and scalable synthesis of polyester and polycarbonate libraries. Journal of the American Chemical Society, 141(22), 8921-8927. https://doi.org/10.1021/jacs.9b02450
Matyjaszewski, K. (2018). Advanced materials by atom transfer radical polymerization. Advanced Materials, 30(23). https://doi.org/10.1002/adma.201706441
Reis, M., Leibfarth, F., & Pitet, L. (2020). Polymerizations in continuous flow: recent advances in the synthesis of diverse polymeric materials. Acs Macro Letters, 9(1), 123-133. https://doi.org/10.1021/acsmacrolett.9b00933
Xiao, Z., Zheng, H., Cheng, D., Zhong, L., Liao, H., Gao, J., … & Wu, Q. (2018). Enhancement on alternating copolymerization of carbon monoxide and styrene by dibenzobarrelene-based α-diimine palladium catalysts. Macromolecules, 51(22), 9110-9121. https://doi.org/10.1021/acs.macromol.8b01786
Boadi, F., Zhang, J., Yu, X., Bhatia, S., & Sampson, N. (2020). Alternating ring-opening metathesis polymerization provides easy access to functional and fully degradable polymers. Macromolecules, 53(14), 5857-5868. https://doi.org/10.1021/acs.macromol.0c01051
Jang, J., Yoon, Y., Jeon, S., Han, Y., & Kim, T. (2021). Molecular weight-dependent, flexible phase behaviors of amphiphilic block copolymer/additive complexes in aqueous solution. Polymers, 13(2), 178. https://doi.org/10.3390/polym13020178
Kai, D., Tan, M., Chee, P., Chua, Y., Yap, Y., & Loh, X. (2016). Towards lignin-based functional materials in a sustainable world. Green Chemistry, 18(5), 1175-1200. https://doi.org/10.1039/c5gc02616d
Nazemi, A., Boott, C., Lunn, D., Gwyther, J., Hayward, D., Richardson, R., … & Manners, I. (2016). Monodisperse cylindrical micelles and block comicelles of controlled length in aqueous media. Journal of the American Chemical Society, 138(13), 4484-4493. https://doi.org/10.1021/jacs.5b13416
Reis, M., Gusev, F., Taylor, N., Chung, S., Verber, M., Lee, Y., … & Leibfarth, F. (2021). Machine learning-guided discovery of 19f mri agents enabled by automated copolymer synthesis.. https://doi.org/10.26434/chemrxiv-2021-rwr0w
Sangroniz, A., Sangroniz, L., Hamzehlou, S., Aranburu, N., Sardón, H., Sarasua, J., … & Etxeberria, A. (2021). Lactide-valerolactone copolymers for packaging applications. Polymers, 14(1), 52. https://doi.org/10.3390/polym14010052
Xia, X., Suzuki, R., Takojima, K., Jiang, D., Isono, T., & Satoh, T. (2021). Smart access to sequentially and architecturally controlled block polymers via a simple catalytic polymerization system. Acs Catalysis, 11(10), 5999-6009. https://doi.org/10.1021/acscatal.1c00382