Synthetic Biology: Innovations, Applications, and Ethical Challenges
This study delves into synthetic biology, focusing on innovative techniques such as CRISPR, therapeutic and biosensing applications, as well as the key ethical and regulatory challenges that are influencing this quickly growing subject.
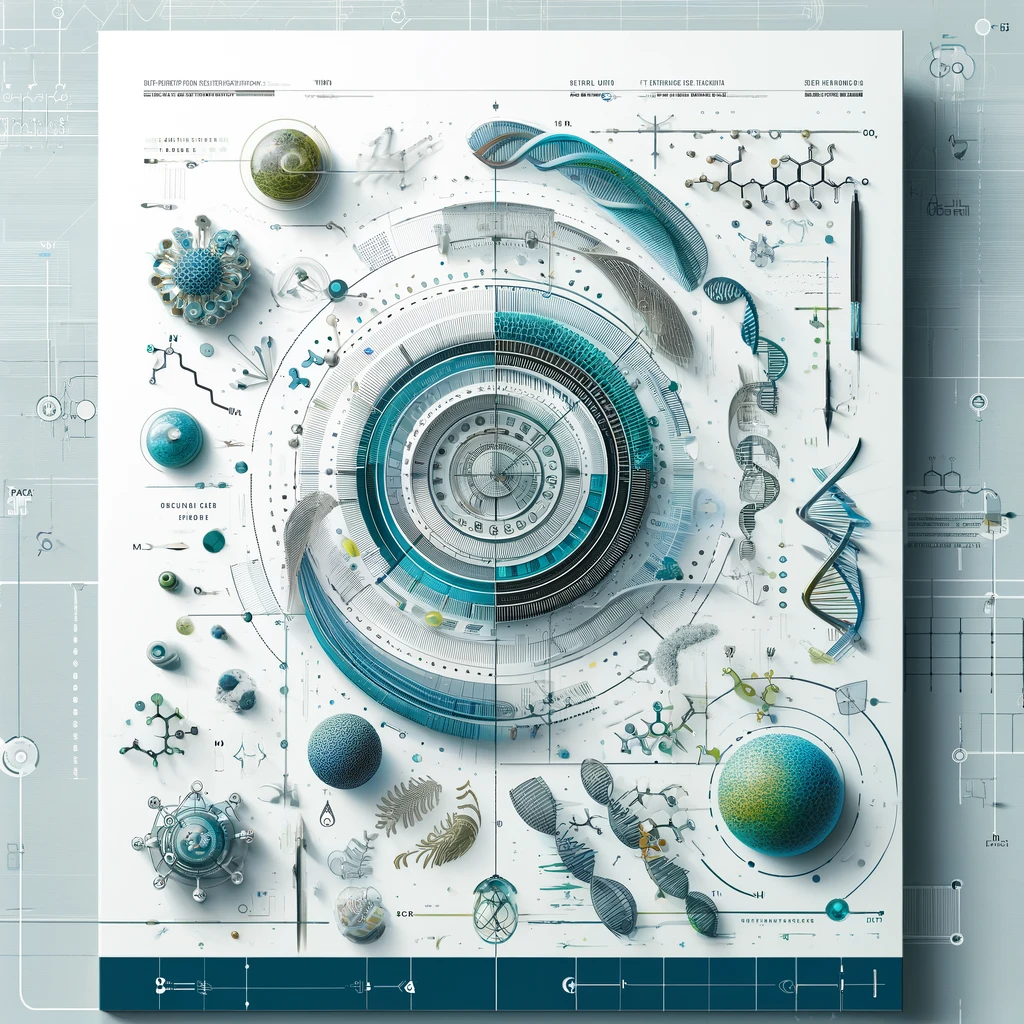
Introduction to Synthetic Biology
Synthetic biology is a field that uses engineering ideas to design and build biological systems for a variety of applications. It has evolved as an important topic in modern science, with the potential for revolutionary advances in fields such as health, biotechnology, and fundamental research (Cheng & Lu, 2012). While the phrase “synthetic biology” has been used since the early twentieth century, it acquired popularity at the turn of the millennium, presenting itself as a fresh and revolutionary discipline (Raimbault et al., 2016). This discipline broadens the scope of genetic engineering to include complete systems of genes and gene products, stressing a holistic approach to biological design (Andrianantoandro et al., 2006).
The history and evolution of synthetic biology have been characterized by a shift toward a more thorough understanding and application of biological systems. Synthetic biology began with genetic engineering, metabolic engineering, and systems biology and has since expanded to include computational biology and bioengineering (Church et al., 2014). Significant advances have been made in the manufacture of biobricks, synthetic cells, genetic circuits, and metabolic pathways, highlighting synthetic biology’s broad uses and potential (Stephanopoulos, 2012).
Furthermore, synthetic biology has the potential to transform several industries, including biomanufacturing and healthcare, by allowing for precise control and modification of biological networks (Rice & Ruder, 2014). The United States has been at the forefront of synthetic biology research, leading the advancement of this burgeoning engineering discipline that creates, controls, and reprograms biological systems (Si & Zhao, 2016). Synthetic biology combines biology and engineering to create programmable artificial systems, enabling novel solutions to challenging challenges (Millar-Haskell et al., 2019).
Synthetic biology is a transformative approach to biological study and engineering that has the potential to alter a wide range of fields by designing and building biological systems based on engineering principles. Synthetic biology’s interdisciplinary character, combining genetics, engineering, and computational biology, puts it as a vital role in defining the future of science and industry.
Core Techniques and Applications
Key strategies in synthetic biology include a variety of creative techniques for designing and building biological systems with specialized functions. Among these approaches, CRISPR technology stands out as an effective tool for precise genetic editing. CRISPR-based synthetic gene circuits have been established to regulate gene expression, control biological processes, and even target specific cells, as evidenced by the detection of bladder cancer cells (Liu et al., 2014). Using CRISPR-Cas9 systems, synthetic gene circuits can be built to serve as logic gates, such as AND gates, allowing for sophisticated control over cellular behavior (Presnell et al., 2022). Furthermore, synthetic biology uses optogenetic methods to build light-responsive genetic circuits, as evidenced in the construction of optogenetic transcription devices for blood-glucose regulation (Ye et al., 2011).
Synthetic gene circuits are important in synthetic biology because they allow for the creation of complicated genomic networks with preset functions. These circuits are intended to process biological signals and perform specialized functions within living cells. Synthetic gene circuits, which incorporate gene regulatory elements like promoters and transcription factors, may conduct logical operations, respond to environmental signals, and regulate gene expression (Nandagopal & Elowitz, 2011). Furthermore, the creation of self-adjusting gene circuits reveals the possibility of rectifying metabolic illnesses by sensing pathological indicators and coordinating therapeutic gene expression (Ye et al., 2016).
In terms of applications, synthetic biology has advanced significantly in medicinal biosynthesis and environmental biosensors. Researchers have used synthetic biology to build microbial hosts for the synthesis of medicinal drugs like paclitaxel using heterologous gene expression (Tong et al., 2021). Furthermore, the development of programmable oncolytic viral immunotherapies for glioblastoma exemplifies the use of synthetic biology principles to create targeted cancer treatments (Monie et al., 2021). Environmental biosensors based on synthetic gene circuits provide novel ways to detect and monitor pollutants, infections, and other environmental variables. Synthetic biology allows for the development of biosensors with greater sensitivity and selectivity by combining gene circuits and microelectronics (Din et al., 2020).
The combination of CRISPR technology, synthetic gene circuits, and optogenetics is a major methodology in synthetic biology, propelling progress in genetic engineering and cellular programming. These approaches have paved the way for a wide range of applications, including therapeutic biosynthesis for drug manufacturing and the development of environmental biosensors to detect biological and chemical signals in the environment.
Ethical and Regulatory Considerations
Ethical concerns about genetic alteration and synthetic life are central to discussions in the field of synthetic biology. The ability to control genetic material and construct synthetic organisms creates difficult ethical issues. Synthetic life, as shown by the production of a bacterial cell controlled by a chemically manufactured genome, has spurred ethical arguments over the status of artificial organisms, the motivations of scientists, and the societal ramifications of such scientific advancements. Gibson, et al. (2010). The ethical implications of synthetic biology are greater than those of classical genetic engineering, necessitating a proactive approach to addressing the ethical norms and professional traditions inherent in transdisciplinary activities (Heidari et al., 2016).
Furthermore, the regulatory landscape in synthetic biology is changing to meet the ethical, safety, and security concerns that come with this developing discipline. Synthetic biology has received policy attention because of societal concerns about life manipulation, economic ramifications, intellectual property rights, environmental impacts, and potential biosecurity hazards (Garfinkel et al., 2007). The European Group on Ethics in Science and New Technologies has addressed the ethical concerns involved with the use of synthetic biology in biosecurity, underlining the dangers of using synthetic pathogens for malicious goals (Jefferson et al., 2014).
Future difficulties in synthetic biology policy include the need for international participation and collaboration to construct global confidence-building measures and address policy options for the engineering biology era (Dixon et al., 2022). The unpredictable and complicated causal webs inherent in synthetic biology present obstacles for addressing ethical and public policy issues, emphasising the significance of finding appropriate approaches to effectively navigate the regulatory landscape (Bedau, 2014). As synthetic biology progresses, it is critical to explore the ethical implications of producing synthetic life forms, the regulatory frameworks that regulate their use, and the societal consequences of these technological advancements.
Reference
Andrianantoandro, E., Basu, S., Karig, D., & Weiss, R. (2006). Synthetic biology: new engineering rules for an emerging discipline. Molecular Systems Biology, 2(1). https://doi.org/10.1038/msb4100073
Cheng, A. and Lu, T. (2012). Synthetic biology: an emerging engineering discipline. Annual Review of Biomedical Engineering, 14(1), 155-178. https://doi.org/10.1146/annurev-bioeng-071811-150118
Church, G., Elowitz, M., Smolke, C., Voigt, C., & Weiss, R. (2014). Realizing the potential of synthetic biology. Nature Reviews Molecular Cell Biology, 15(4), 289-294. https://doi.org/10.1038/nrm3767
Millar‐Haskell, C., Dang, A., & Gleghorn, J. (2019). Coupling synthetic biology and programmable materials to construct complex tissue ecosystems. Mrs Communications, 9(2), 421-432. https://doi.org/10.1557/mrc.2019.69
Raimbault, B., Cointet, J., & Joly, P. (2016). Mapping the emergence of synthetic biology. Plos One, 11(9), e0161522. https://doi.org/10.1371/journal.pone.0161522
Rice, M. and Ruder, W. (2014). Creating biological nanomaterials using synthetic biology. Science and Technology of Advanced Materials, 15(1), 014401. https://doi.org/10.1088/1468-6996/15/1/014401
Si, T. and Zhao, H. (2016). A brief overview of synthetic biology research programs and roadmap studies in the united states. Synthetic and Systems Biotechnology, 1(4), 258-264. https://doi.org/10.1016/j.synbio.2016.08.003
Stephanopoulos, G. (2012). Synthetic biology and metabolic engineering. Acs Synthetic Biology, 1(11), 514-525. https://doi.org/10.1021/sb300094q
Din, M., Martin, A., Razinkov, I., Csicsery, N., & Hasty, J. (2020). Interfacing gene circuits with microelectronics through engineered population dynamics. Science Advances, 6(21). https://doi.org/10.1126/sciadv.aaz8344
Liu, Y., Zeng, Y., Liu, L., Zhuang, C., Fu, X., Huang, W., … & Cai, Z. (2014). Synthesizing and gate genetic circuits based on crispr-cas9 for identification of bladder cancer cells. Nature Communications, 5(1). https://doi.org/10.1038/ncomms6393
Monie, D., Bhandarkar, A., Parney, I., Correia, C., Sarkaria, J., Vile, R., … & Hu, L. (2021). Synthetic and systems biology principles in the design of programmable oncolytic virus immunotherapies for glioblastoma. Neurosurgical Focus, 50(2), E10. https://doi.org/10.3171/2020.12.focus20855
Nandagopal, N. and Elowitz, M. (2011). Synthetic biology: integrated gene circuits. Science, 333(6047), 1244-1248. https://doi.org/10.1126/science.1207084
Presnell, K., Melhem, O., Morse, N., & Alper, H. (2022). Modular, synthetic boolean logic gates enabled in saccharomyces cerevisiae through t7 polymerases/crispr dcas9 designs. Acs Synthetic Biology, 11(10), 3414-3425. https://doi.org/10.1021/acssynbio.2c00327
Tong, Y., Luo, Y., & Gao, W. (2021). Biosynthesis of paclitaxel using synthetic biology. Phytochemistry Reviews, 21(3), 863-877. https://doi.org/10.1007/s11101-021-09766-0
Ye, H., Baba, M., Peng, R., & Fussenegger, M. (2011). A synthetic optogenetic transcription device enhances blood-glucose homeostasis in mice. Science, 332(6037), 1565-1568. https://doi.org/10.1126/science.1203535
Ye, H., Xie, M., Xue, S., Hamri, G., Yin, J., Zulewski, H., … & Fussenegger, M. (2016). Self-adjusting synthetic gene circuit for correcting insulin resistance. Nature Biomedical Engineering, 1(1). https://doi.org/10.1038/s41551-016-0005
Bedau, M. (2014). Policy‐making and systemic complexity. The Hastings Center Report, 44(S5). https://doi.org/10.1002/hast.394
Dixon, T., Freemont, P., Johnson, R., & Pretorius, I. (2022). A global forum on synthetic biology: the need for international engagement. Nature Communications, 13(1). https://doi.org/10.1038/s41467-022-31265-9
Garfinkel, M., Endy, D., Epstein, G., & Friedman, R. (2007). Synthetic genomics | options for governance. Biosecurity and Bioterrorism Biodefense Strategy Practice and Science, 5(4), 359-362. https://doi.org/10.1089/bsp.2007.0923
Gibson, D., Glass, J., Lartigue, C., Noskov, V., Chuang, R., Algire, M., … & Venter, J. (2010). Creation of a bacterial cell controlled by a chemically synthesized genome. Science, 329(5987), 52-56. https://doi.org/10.1126/science.1190719
Heidari, R., Elger, B., & Stutzki, R. (2016). On the brink of shifting paradigms, molecular systems engineering ethics needs to take a proactive approach. Chimia International Journal for Chemistry, 70(6), 449. https://doi.org/10.2533/chimia.2016.449
Jefferson, C., Lentzos, F., & Marris, C. (2014). Synthetic biology and biosecurity: challenging the myths. Frontiers in Public Health, 2. https://doi.org/10.3389/fpubh.2014.00115