Recent Advances in Algae Chemistry
Algae chemistry is revolutionizing medications, environmental cleanup, and sustainable biofuels. Recent scientific findings and technology innovations are transforming algae for a cleaner future.
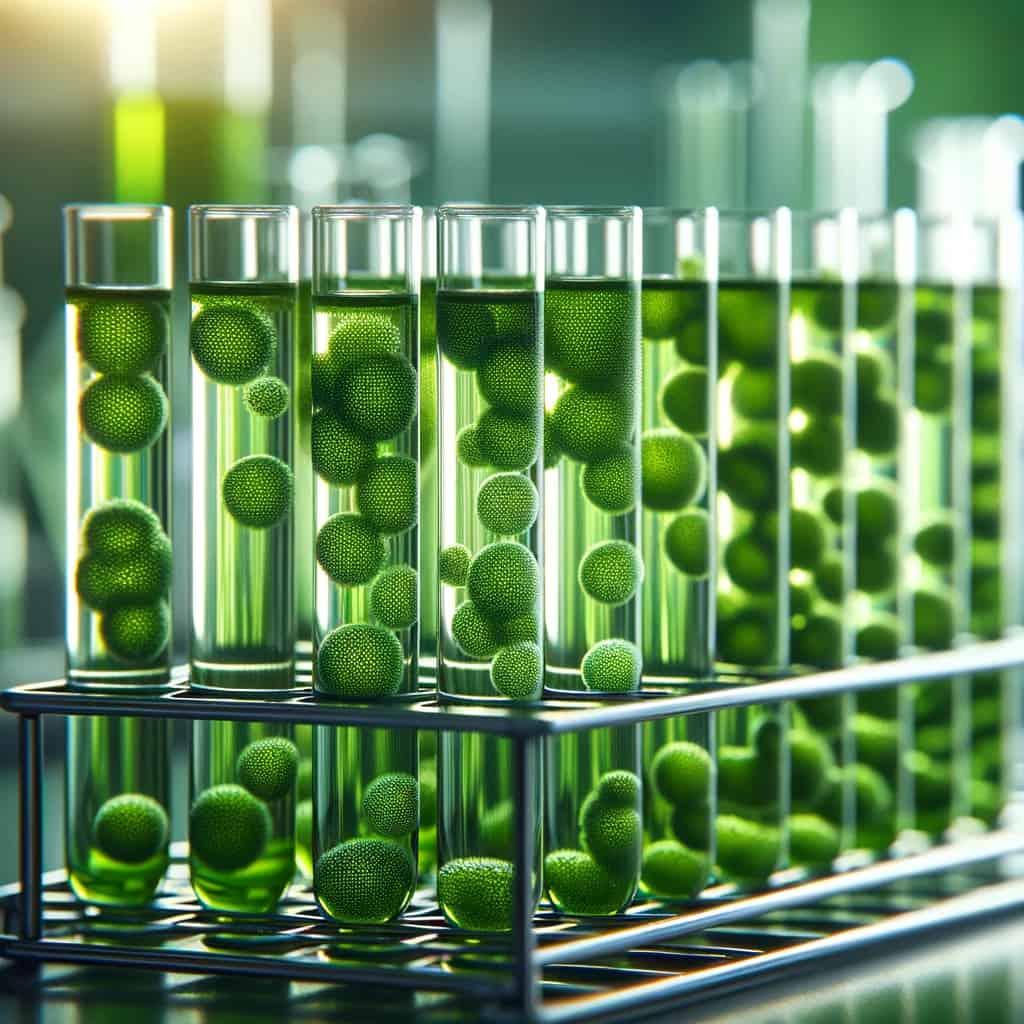
I. Introduction to Algal Chemistry
A wide range of freshwater, marine, and even terrestrial settings are home to the varied group of autotrophic organisms known as algae (Salami et al., 2021). With species ranging from unicellular to multicellular forms, they show a significant degree of morphological variation (Misumi et al., 2007). According to Tanabe et al. (2011), algae have evolved to live in a variety of ecological niches, including freshwater, subaerial, and even harsh settings like the snow surfaces seen in alpine locations. Certain types of algae, like calcareous algae, are known to grow best in particular conditions; for example, green algae like warm waters, but red algae can grow anywhere in the world (Basso & Granier, 2012).
Algae’s principal chemical components are essential to its biological processes. Many pigments, including phycobilins, carotenoids, and chlorophylls, are found in algae and are necessary for photosynthesis and light absorption (Shalaby, 2011). The various colors seen in various algae species are a result of these pigments. Lipids are also found in algae, especially long-chain omega-3 fatty acids, which are crucial for membrane construction and energy storage (Hori et al., 2014). Algae include large amounts of polysaccharides including alginate, carrageenan, and cellulose, which act as structural elements and energy stores (Shalaby, 2011). Algal proteins also participate in a variety of cellular functions and defensive mechanisms (Shalaby, 2011).
Algae have a special process called photosynthesis that is different from that of terrestrial plants in certain ways. Algae may flourish in a variety of settings because they have developed effective photosynthetic processes. Algae have a major role in primary production, particularly those found in marine environments (Røkke et al., 2019). Algae have unique photosynthetic systems that improve light gathering and energy transfer efficiency even under low-light conditions. Red algae, for example, feature phycobilisomes (Xie et al., 2021). Due to these changes, algae may successfully photosynthesize in a variety of lighting conditions, including low light (Xie et al., 2021).
Algae are a broad collection of creatures that have a wide range of environmental adaptations, to sum up. Their main chemical components—proteins, lipids, polysaccharides, and pigments—are crucial to their biological processes. Algae are essential components of ecosystems all over the world because they have developed special photosynthetic mechanisms that are effective and adapted to their particular environments.
II. Applications of Algal Chemistry
The manufacture of biofuels, especially biodiesel made from algae oil, is greatly aided by algae. Because of their high lipid content and quick growth rates, algae are thought to be a promising feedstock for the generation of biodiesel (Greenwell et al., 2009). Algal oil’s physicochemical characteristics play a critical role in deciding whether or not it is suitable for the production of biodiesel (Laurelta & Oghenekaro, 2023). The goal of research has been to increase the economic viability of producing microalgal biodiesel by advancing technology (Szulczyk et al., 2022). For the manufacture of commercial biodiesel, choosing the right algae species is crucial, taking into account aspects like oil yield and quality (Tiwari et al., 2021). Research has also looked into improving the transesterification parameters to increase the productivity of algal biodiesel production (Murthy & Kumar, 2021). International criteria for automobile use have been determined to be met by algal biodiesel, which has similar physical and chemical properties to conventional diesel (PRAJAPATI & BARAIYA, 2023). Moreover, life cycle analyses have been carried out to appraise the energy needs and ecological consequences of algae biodiesel manufacturing systems (Ponnusamy et al., 2014).
Algae are a rich source of bioactive chemicals with a variety of health advantages that are used in the pharmaceutical and nutraceutical sectors. Algae have anti-inflammatory, antioxidant, and vitamin properties that may have medical uses (Greenwell et al., 2009). The increasing interest in algae as a source of useful components for goods that promote health is largely due to these bioactive chemicals (Greenwell et al., 2009).
The food sector uses algae extensively for a variety of reasons. Because polysaccharides like alginate and carrageenan are present, they act as thickening agents in food products. Popular dietary supplements, algae like spirulina and chlorella are prized for their high nutritional content, which includes vital elements including proteins, vitamins, and minerals (Greenwell et al., 2009). Furthermore, algae provide a vegan alternative to fish-derived omega-3 supplements by serving as a sustainable source of these fatty acids (Greenwell et al., 2009).
Furthermore, algae are essential to efforts to clean up the environment. Through photosynthesis, they aid in the capture of carbon, reducing greenhouse gas emissions and battling climate change (Greenwell et al., 2009). In order to improve water quality and the health of ecosystems, algae are also employed in wastewater treatment processes to remove contaminants like phosphorus and nitrogen (Greenwell et al., 2009). Algae are useful in environmental cleanup projects because they can take up nutrients and pollutants from water.
Algae are adaptable creatures with uses in a range of sectors, to sum up. Algae are a rich and sustainable resource with a range of health and environmental benefits, including biofuels, food, medicines, and environmental remediation.
III. Challenges and Future Directions
There are a number of difficulties in growing algae at a sustainable and profitable scale. To maximize algal production on a wide scale, concerns like contamination, water utilization, and harvesting efficiency must be resolved. Since the process of growing algae requires a lot of water, water scarcity is a major worry. To reduce the negative effects on the ecosystem, it is essential to balance water usage with sustainable methods Clarens et al. (2010). Unwanted bacteria can impede the growth of algal cultures and degrade the quality of the biomass they produce. Successful cultivation requires the development of ways to maintain the purity of algal cultures and prevent contamination (Fulbright et al., 2018). Efficiently harvesting algae poses a challenge due to their small size and negative charge, which makes them challenging to separate from the growth media. Large-scale production depends on improving harvesting methods to boost productivity and lower energy usage (Li et al., 2021).
New developments in technology present encouraging ways to address current difficulties in the processing and growth of algae. Separation technology advancements may increase the effectiveness of removing algae from the growing medium while lowering expenses and energy usage (Wiley et al., 2011). In order to maximize the growing conditions for algae and increase productivity and biomass generation, advances in photobioreactor design and operation are being made (Narala et al., 2016). Furthermore, the advancement of natural coagulants and self-flocculation techniques for the harvesting of algae indicates promise for raising the effectiveness of biomass recovery (Amr et al., 2022). By using wastewater nutrients for algal growth, the integration of algal farming with wastewater treatment procedures can improve sustainability (Deng et al., 2018). Additionally, anaerobic digestate and recycled media can reduce the need for commercial fertilizers in algal culture systems, increasing the sustainability of output (Xinyi et al., 2016).
The uncharted territory of algae chemistry presents promising opportunities for the discovery of novel bioactive substances with a range of uses. The enormous diversity of algae species that are still unexplored offers a chance to find novel chemicals with industrial, medicinal, and nutraceutical potential. Although algae are known to be a possible source of vitamins, antioxidants, and anti-inflammatory agents, more research into their chemical makeup may reveal other bioactive substances that are beneficial to human health (Diaz et al., 2023). Innovative methods for boosting algal growth and productivity may result from research into the allelopathic qualities of algae and their potential to enhance cultivation techniques (Mendes & Vermelho, 2013).
Although extensive algal production has the potential to be sustainable, the effects on the environment must be carefully considered. To ensure long-term sustainability, algal production systems need to be built with the least amount of water and nutrients depleted possible (Beal et al., 2012). It is imperative to closely monitor and manage any potential negative effects of algal production on nearby ecosystems, such as alterations in water quality and biodiversity (Prasad, 2015). Using algae’s capacity to absorb nutrients and pollutants from water, phytoremediation techniques can aid in environmental remediation efforts (Guleri et al., 2020). The environmental impact of algal production can be decreased by combining algae culture with wastewater treatment procedures, leading to a more sustainable method of producing biomass and biofuel (Houser et al., 2014).
In conclusion, overcoming the difficulties associated with large-scale algae cultivation calls for a multidisciplinary strategy that incorporates environmental stewardship, sustainable practices, and technical innovation. There is hope for the future of large-scale algal production to meet a variety of societal needs while protecting ecosystems by utilizing recent advancements in algal cultivation technologies, investigating the unexplored potential of algal chemistry, and giving sustainability and environmental impact assessments top priority.
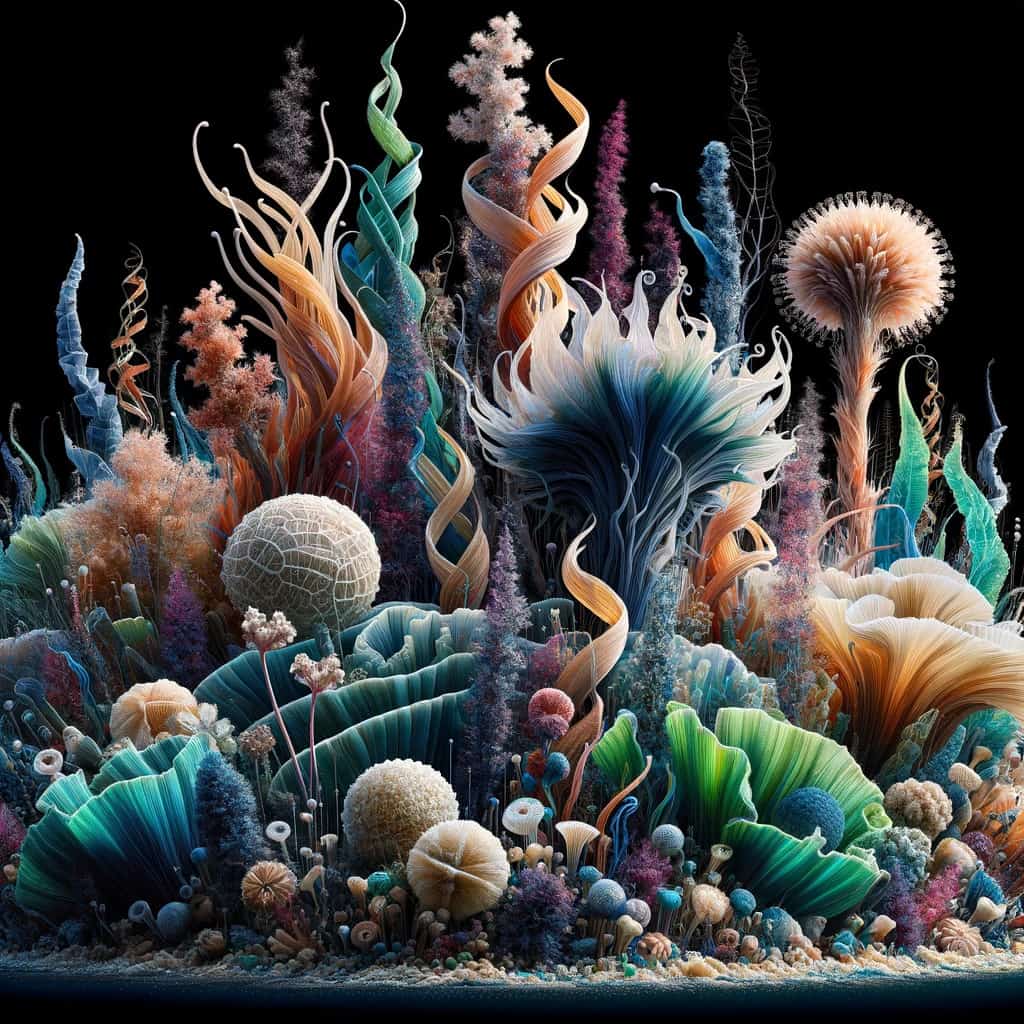
Reference:
Amr, S., Abujazar, M., Alazaiza, M., Albahnasawi, A., Bashir, M., & Nassani, D. (2022). The potential use of natural coagulants for microalgae harvesting: a review. Water Quality Research Journal, 58(1), 54-74. https://doi.org/10.2166/wqrj.2022.026
Basso, D. and Granier, B. (2012). Calcareous algae in changing environments. Geodiversitas, 34(1), 5-11. https://doi.org/10.5252/g2012n1a1
Beal, C., Stillwell, A., King, C., Cohen, S., Berberoğlu, H., Bhattarai, R., … & Hebner, R. (2012). Energy return on investment for algal biofuel production coupled with wastewater treatment. Water Environment Research, 84(9), 692-710. https://doi.org/10.2175/106143012×13378023685718
Clarens, A., Resurreccion, E., White, M., & Colosi, L. (2010). Environmental life cycle comparison of algae to other bioenergy feedstocks. Environmental Science & Technology, 44(5), 1813-1819. https://doi.org/10.1021/es902838n
Deng, X., Gao, K., Addy, M., Chen, P., Da, L., Zhang, R., … & Ruan, R. (2018). Growing chlorella vulgaris on mixed wastewaters for biodiesel feedstock production and nutrient removal. Journal of Chemical Technology & Biotechnology, 93(9), 2748-2757. https://doi.org/10.1002/jctb.5634
Diaz, C., Douglas, K., Kang, K., Kolarik, A., Malinovski, R., Torres-Tiji, Y., … & Mayfield, S. (2023). Developing algae as a sustainable food source. Frontiers in Nutrition, 9. https://doi.org/10.3389/fnut.2022.1029841
Fulbright, S., Robbins‐Pianka, A., Berg-Lyons, D., Knight, R., Reardon, K., & Chisholm, S. (2018). Bacterial community changes in an industrial algae production system. Algal Research, 31, 147-156. https://doi.org/10.1016/j.algal.2017.09.010
Greenwell, H., Laurens, L., Shields, R., Lovitt, R., & Flynn, K. (2009). Placing microalgae on the biofuels priority list: a review of the technological challenges. Journal of the Royal Society Interface, 7(46), 703-726. https://doi.org/10.1098/rsif.2009.0322
Guleri, S., Singh, K., Kaushik, R., Dhankar, R., & Tiwari, A. (2020). Phycoremediation: a novel and synergistic approach in wastewater remediation. Journal of Microbiology Biotechnology and Food Sciences, 10(1), 98-106. https://doi.org/10.15414/jmbfs.2020.10.1.98-106
Hori, K., Maruyama, F., Fujisawa, T., Togashi, T., Yamamoto, N., Seo, M., … & Ohta, H. (2014). Klebsormidium flaccidum genome reveals primary factors for plant terrestrial adaptation. Nature Communications, 5(1). https://doi.org/10.1038/ncomms4978
Houser, J., Venable, M., Sakamachi, Y., Hambourger, M., Herrin, J., & Tuberty, S. (2014). Wastewater remediation using algae grown on a substrate for biomass and biofuel production. Journal of Environmental Protection, 05(10), 895-904. https://doi.org/10.4236/jep.2014.510091
Laurelta, T. and Oghenekaro, I. (2023). An assessment of biodiesel production from three species of micro algae (chlamydomonas reinhardtii, chroococcus species and ankistrodesmus falcatus). Journal of Advances in Biology & Biotechnology, 26(2), 9-20. https://doi.org/10.9734/jabb/2023/v26i2618
Li, T., Hu, J., & Zhu, L. (2021). Self-flocculation as an efficient method to harvest microalgae: a mini-review. Water, 13(18), 2585. https://doi.org/10.3390/w13182585
Mendes, L. and Vermelho, A. (2013). Allelopathy as a potential strategy to improve microalgae cultivation. Biotechnology for Biofuels, 6(1), 152. https://doi.org/10.1186/1754-6834-6-152
Misumi, O., Yoshida, Y., Nishida, K., Fujiwara, T., Sakajiri, T., Hirooka, S., … & Kuroiwa, T. (2007). Genome analysis and its significance in four unicellular algae, cyanidioshyzon merolae, ostreococcus tauri, chlamydomonas reinhardtii, and thalassiosira pseudonana. Journal of Plant Research, 121(1), 3-17. https://doi.org/10.1007/s10265-007-0133-9
Murthy, G. and Kumar, R. (2021). Chemical characterization of algae oil and optimization of transesterification parameters for boosting of algal biodiesel production from spirulina wild stuff explored in the natural habitation. Egyptian Journal of Chemistry, 0(0), 0-0. https://doi.org/10.21608/ejchem.2021.51764.3104
Narala, R., Garg, S., Sharma, K., Thomas‐Hall, S., Deme, M., Li, Y., … & Schenk, P. (2016). Comparison of microalgae cultivation in photobioreactor, open raceway pond, and a two-stage hybrid system. Frontiers in Energy Research, 4. https://doi.org/10.3389/fenrg.2016.00029
PRAJAPATI, R. and BARAIYA, M. (2023). Production of biodiesel from natural algae. Interantional Journal of Scientific Research in Engineering and Management, 07(04). https://doi.org/10.55041/ijsrem18755
Ponnusamy, S., Reddy, H., Muppaneni, T., Downes, C., & Deng, S. (2014). Life cycle assessment of biodiesel production from algal bio-crude oils extracted under subcritical water conditions. Bioresource Technology, 170, 454-461. https://doi.org/10.1016/j.biortech.2014.07.072
Prasad, M. (2015). Phytoremediation crops and biofuels., 159-261. https://doi.org/10.1007/978-3-319-16742-8_7
Røkke, G., Melø, T., Vadstein, O., Bones, A., & Hohmann-Marriott, M. (2019). Unique photosynthetic electron transport tuning and excitation distribution in heterokont algae. Plos One, 14(1), e0209920. https://doi.org/10.1371/journal.pone.0209920
Salami, R., Kordi, M., Bolouri, P., Delangiz, N., & Lajayer, B. (2021). Algae-based biorefinery as a sustainable renewable resource. Circular Economy and Sustainability, 1(4), 1349-1365. https://doi.org/10.1007/s43615-021-00088-z
Shalaby, E. (2011). Algae as promising organisms for environment and health. Plant Signaling & Behavior, 6(9), 1338-1350. https://doi.org/10.4161/psb.6.9.16779
Szulczyk, K., Cheema, M., & Ziaei, S. (2022). Could technological improvements make microalgal biodiesel more economically feasible?. Iop Conference Series Earth and Environmental Science, 1074(1), 012006. https://doi.org/10.1088/1755-1315/1074/1/012006
Tanabe, Y., Shitara, T., Kashino, Y., Hara, Y., & Kudoh, S. (2011). Utilizing the effective xanthophyll cycle for blooming of ochromonas smithii and o. itoi (chrysophyceae) on the snow surface. Plos One, 6(2), e14690. https://doi.org/10.1371/journal.pone.0014690
Tiwari, V., Das, A., Thakur, S., & Trivedi, R. (2021). Selection of indigenous algal species for potential biodiesel production. Journal of Pure and Applied Microbiology, 15(2), 851-863. https://doi.org/10.22207/jpam.15.2.40
Wiley, P., Campbell, J., & McKuin, B. (2011). Production of biodiesel and biogas from algae: a review of process train options. Water Environment Research, 83(4), 326-338. https://doi.org/10.2175/106143010×12780288628615
Xie, M., Li, W., Lin, H., Wang, X., Dong, J., Qin, S., … & Zhao, F. (2021). Difference in light use strategy in red alga between griffithsia pacifica and porphyridium purpureum. Scientific Reports, 11(1). https://doi.org/10.1038/s41598-021-93696-6
Xinyi, E., Crofcheck, C., & Crocker, M. (2016). Application of recycled media and algae-based anaerobic digestate in scenedesmus cultivation. Journal of Renewable and Sustainable Energy, 8(1). https://doi.org/10.1063/1.4942782