Parkinson’s Disease: Chemical Insights & Innovations
This article investigates the chemical basis of Parkinson’s disease (PD). It assesses current chemical-based treatments, and dives into potential research areas including gene and stem cell therapy.
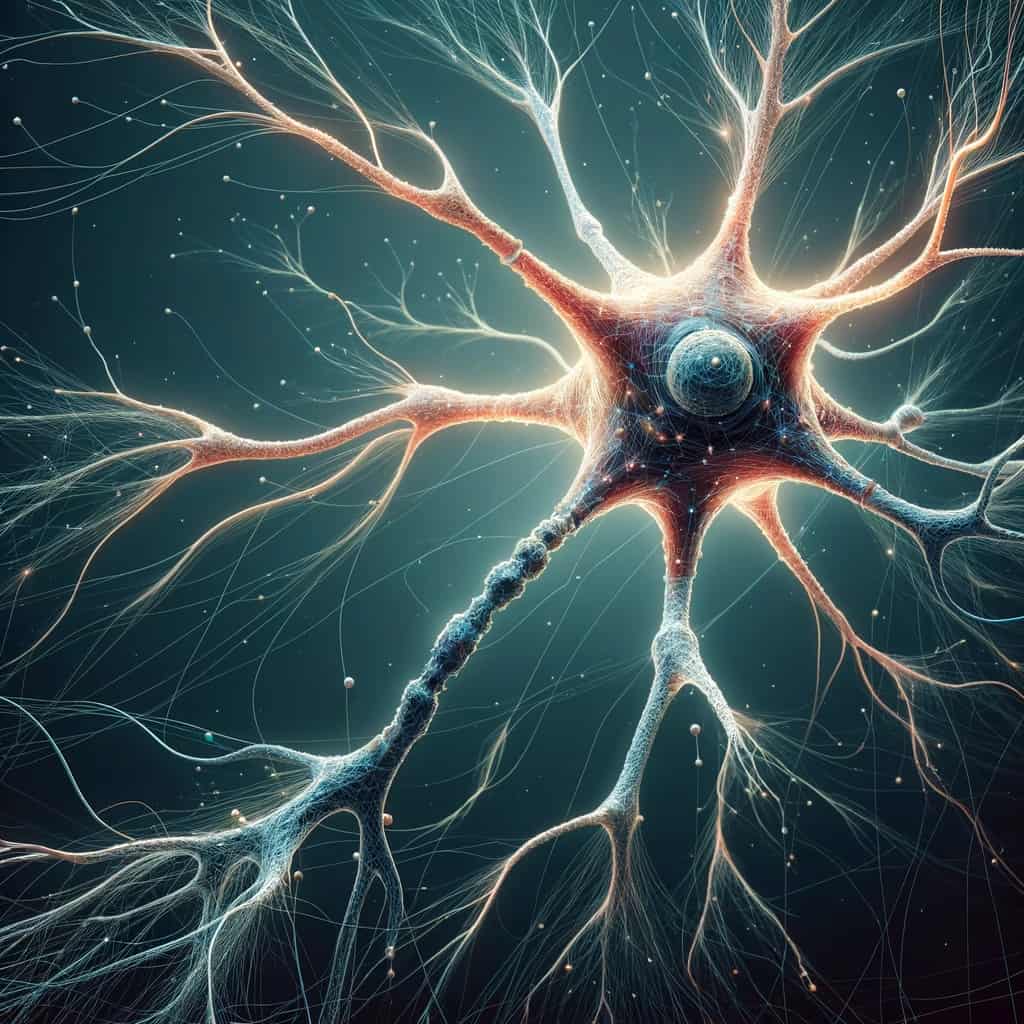
Introduction to Parkinson’s Disease and Its Chemical Underpinnings
Parkinson’s disease (PD) is a neurodegenerative disorder defined by the progressive loss of dopaminergic neurons in the substantia nigra, resulting in a severe reduction in dopamine levels in the brain. The substantia nigra is an important location for motor control, and the loss of dopamine-producing cells in this area contributes to motor symptoms seen in Parkinson’s disease, such as tremors, muscle rigidity, and decreased balance (Chinta & Andersen, 2008; Ikeda et al., 2008). The drop in dopamine levels affects the delicate balance of neurotransmitters in crucial brain circuits, especially the interaction between glutamate and dopamine in the striatum, which is required for motor control (Zhang et al., 2014). Parkinson’s disease psychosis (PDP) is hypothesized to be caused by a neurotransmitter imbalance, specifically a disruption of the usual balance between the serotonergic and dopaminergic systems (Stahl, 2016).
In addition to motor symptoms, Parkinson’s disease is associated with cognitive impairment, impacting attention, memory, and executive function (Elgh et al., 2009). Furthermore, postural imbalance and gait abnormalities are common characteristics of Parkinson’s disease (Fukunaga et al., 2014). Posturography has been proposed as a way for objectively assessing imbalance in Parkinson’s disease patients, reflecting the clinical disequilibrium found in these individuals (Ebersbach and Gunkel, 2010).
The importance of oxidative stress in Parkinson’s disease pathogenesis is well established, with studies demonstrating the impact of redox imbalance and mitochondrial dysfunction on disease progression (Double et al., 2019; Pérez-Carrión et al., 2022). Oxidative stress causes the production of free radicals, which can harm cells and contribute to the degeneration of dopaminergic neurons in the substantia nigra (Double et al. 2019). Furthermore, the presence of insoluble protein aggregates known as Lewy bodies is a hallmark of Parkinson’s disease pathology, showing protein misfolding and aggregation events in the brain (Pérez-Carrión et al., 2022).
A variety of therapy techniques have been investigated to address the chemical imbalances associated with Parkinson’s disease. For example, Tanabe et al. (2014) explored the impact of tyrosine kinase inhibitors on restoring striatal motor behaviors in animal models of Parkinson’s disease. Furthermore, the transplantation of GABAergic interneurons has showed promise in lowering Parkinsonian-like motor abnormalities, underlining the possibility of cell-based therapies in treating neurotransmitter imbalances in Parkinson’s disease (Southwell et al., 2014).
The drop in dopamine levels and the ensuing chemical imbalances in the brain are critical in the pathogenesis of Parkinson’s disease, contributing to a variety of motor and non-motor symptoms. Understanding these chemical underpinnings is critical for developing successful Parkinson’s disease therapies.
Chemical Targets for Parkinson’s Disease Treatment
Parkinson’s Disease (PD) treatment frequently focuses on chemical imbalances in the brain, specifically dopamine levels. Levodopa and other dopamine precursors play an important role in treating Parkinson’s disease symptoms by replenishing dopamine levels in the brain. Lee et al. (1999); Chen et al. (2008). Levodopa is regarded the gold standard treatment for Parkinson’s disease because it successfully resolves the dopamine shortage that causes motor symptoms such as tremors and muscle stiffness (Hershey et al., 1998; Sawant et al., 2019). However, levodopa medication might cause difficulties such as dyskinesias over time (Sawant et al., 2019).
Monoamine oxidase B (MAO-B) inhibitors are another type of medicine used to treat Parkinson’s disease. MAO-B inhibitors limit dopamine breakdown in the brain, raising dopamine levels and alleviating motor symptoms (Hu et al., 2012; Tang et al., 2019). These inhibitors are especially effective in the early stages of Parkinson’s disease, when dopamine-producing neurons are still active (Marino et al., 2003).
In addition to dopamine precursors and MAO-B inhibitors, various neuroprotective medicines are being investigated for their potential use in Parkinson’s disease treatment. These medicines are designed to protect dopaminergic neurons from degeneration and oxidative stress, both of which are common hallmarks of Parkinson’s disease (Lee et al., 2019; Shook & Jackson, 2011). For example, thyroid hormone derivatives have showed promise in stimulating dopamine neuron differentiation, suggesting a potential neuroprotective therapy for Parkinson’s disease (Shook & Jackson, 2011).
Furthermore, dopamine receptor and neurotransmitter system modification is being studied as a treatment for Parkinson’s disease. Allosteric modulation of metabotropic glutamate receptors and adenosine A2A receptor antagonists are being studied as potential treatments for Parkinson’s disease via modulating dopamine signaling in the brain (Lee et al., 2019). These treatments aim to fine-tune dopamine transmission and improve motor function in Parkinson’s disease patients.
Current Parkinson’s disease treatments aim to correct chemical imbalances in the brain, with a primary focus on refilling dopamine levels, avoiding dopamine breakdown, and investigating neuroprotective techniques to manage motor and non-motor symptoms. While dopamine replacement medication remains a cornerstone of PD management, continuing research into innovative therapeutic targets and techniques attempts to improve treatment efficacy while also addressing the disease’s complicated pathogenesis.
Emerging Chemical Therapies and Research Directions
Cutting-edge research into chemical treatments for Parkinson’s disease (PD) is paving the way for novel therapeutic options that aim to reduce disease progression and improve patient outcomes. Gene therapy, stem cell therapy, and novel pharmacological techniques are at the forefront of these breakthroughs, providing significant opportunities for the development of disease-modifying therapeutics.
Gene therapy has significant potential for treating Parkinson’s disease, with research focusing on delivering therapeutic genes to disease-affected brain areas. Albert et al. (2017) conducted research on the use of adeno-associated virus (AAV) vectors for gene delivery to substantia nigra dopamine neurons in order to induce the synthesis of neurotrophic factors for disease-modifying therapies. Furthermore, research have looked into the possibility of replacing tyrosine hydroxylase using transvascular gene therapy as a novel approach to Parkinson’s disease treatment (Pardridge, 2005). These gene therapy options target key pathways implicated in Parkinson’s disease development, with the potential for long-term treatment advantages.
Stem cell therapy is another cutting-edge method to Parkinson’s disease treatment. Researchers hope to restore normal brain function by replacing injured dopaminergic neurons with neuronal stem cells generated from bone marrow stem cells (Zhang et al., 2007). This method has the potential to regenerate dopaminergic neurons and delay the course of Parkinson’s disease.
Novel pharmaceutical methods are also being explored to target particular molecular processes associated with Parkinson’s disease. For example, research of the glucocerebrosidase gene found a probable link with Parkinson’s disease, implying that changes in this gene may contribute to disease vulnerability (Sato et al. 2005). Furthermore, supplementary integrative techniques, such as acupuncture therapy, have showed promise in treating Parkinson’s disease symptoms and improving patients’ quality of life (Shulman et al., 2002).
Understanding the chemical basis of Parkinson’s disease is critical for the development of future therapies. Researchers can develop more effective treatments for Parkinson’s disease by targeting particular biological pathways implicated in the disease rather than simply controlling symptoms. The combination of gene therapy, stem cell therapy, and innovative pharmaceutical methods provides a complex strategy for slowing Parkinson’s disease development and improving patient outcomes.
In conclusion, continuous research into cutting-edge chemical therapeutics for Parkinson’s disease emphasizes the necessity of novel techniques in generating disease-modifying medications. Researchers are working to develop more specific and effective medicines that have the potential to reduce disease progression and enhance the quality of life for people with Parkinson’s disease by utilizing gene therapy, stem cell therapy, and innovative pharmaceutical techniques.
Reference:
Albert, K., Voutilainen, M., Domanskyi, A., & Airavaara, M. (2017). Aav vector-mediated gene delivery to substantia nigra dopamine neurons: implications for gene therapy and disease models. Genes, 8(2), 63. https://doi.org/10.3390/genes8020063
Chen, L., Ding, Y., Cagniard, B., Laar, A., Mortimer, A., Chi, W., … & Zhuang, X. (2008). Unregulated cytosolic dopamine causes neurodegeneration associated with oxidative stress in mice. Journal of Neuroscience, 28(2), 425-433. https://doi.org/10.1523/jneurosci.3602-07.2008
Chinta, S. and Andersen, J. (2008). Redox imbalance in parkinson’s disease. Biochimica Et Biophysica Acta (Bba) – General Subjects, 1780(11), 1362-1367. https://doi.org/10.1016/j.bbagen.2008.02.005
Double, K., Hare, D., & Double, K. (2019). Oxidative stress in the aging substantia nigra and the etiology of parkinson’s disease. Aging Cell, 18(6). https://doi.org/10.1111/acel.13031
Ebersbach, G. and Gunkel, M. (2010). Posturography reflects clinical imbalance in parkinson’s disease. Movement Disorders, 26(2), 241-246. https://doi.org/10.1002/mds.23189
Elgh, E., Domellöf, M., Lindér, J., Edström, M., Stenlund, H., & Forsgren, L. (2009). Cognitive function in early parkinson’s disease: a population‐based study. European Journal of Neurology, 16(12), 1278-1284. https://doi.org/10.1111/j.1468-1331.2009.02707.x
Fukunaga, J., Quitschal, R., Doná, F., Ferraz, H., Ganança, M., & Caovilla, H. (2014). Postural control in parkinson’s disease. Brazilian Journal of Otorhinolaryngology, 80(6), 508-514. https://doi.org/10.1016/j.bjorl.2014.05.032
Hershey, T., Black, K., Stambuk, M., Carl, J., McGee-Minnich, L., & Perlmutter, J. (1998). Altered thalamic response to levodopa in parkinson’s patients with dopa-induced dyskinesias. Proceedings of the National Academy of Sciences, 95(20), 12016-12021. https://doi.org/10.1073/pnas.95.20.12016
Hu, S., Nian, S., Qin, K., Xiao, T., Li, L., Qi, X., … & Song, B. (2012). Design, synthesis and inhibitory activities of 8-(substituted styrol-formamido)phenyl-xanthine derivatives on monoamine oxidase b. Chemical and Pharmaceutical Bulletin, 60(3), 385-390. https://doi.org/10.1248/cpb.60.385
Ikeda, Y., Tsuji, S., Satoh, A., Ishikura, M., Shirasawa, T., & Shimizu, T. (2008). Protective effects of astaxanthin on 6‐hydroxydopamine‐induced apoptosis in human neuroblastoma sh‐sy5y cells. Journal of Neurochemistry, 107(6), 1730-1740. https://doi.org/10.1111/j.1471-4159.2008.05743.x
Lee, E., Kim, S., Kim, C., Pagire, S., Pagire, H., Chung, H., … & Park, C. (2019). Dopamine neuron induction and the neuroprotective effects of thyroid hormone derivatives. Scientific Reports, 9(1). https://doi.org/10.1038/s41598-019-49876-6
Lee, W., Chang, J., Nemeth, N., & Kang, U. (1999). Vesicular monoamine transporter-2 and aromaticl-amino acid decarboxylase enhance dopamine delivery afterl-3,4-dihydroxyphenylalanine administration in parkinsonian rats. Journal of Neuroscience, 19(8), 3266-3274. https://doi.org/10.1523/jneurosci.19-08-03266.1999
Marino, M., Williams, D., O’Brien, J., Valenti, O., McDonald, T., Clements, M., … & Conn, P. (2003). Allosteric modulation of group iii metabotropic glutamate receptor 4: a potential approach to parkinson’s disease treatment. Proceedings of the National Academy of Sciences, 100(23), 13668-13673. https://doi.org/10.1073/pnas.1835724100
Pardridge, W. (2005). Tyrosine hydroxylase replacement in experimental parkinson’s disease with transvascular gene therapy. Neurorx, 2(1), 129-138. https://doi.org/10.1602/neurorx.2.1.129
Pérez-Carrión, M., Posadas, I., Solera, J., & Ceña, V. (2022). Lrrk2 and proteostasis in parkinson’s disease. International Journal of Molecular Sciences, 23(12), 6808. https://doi.org/10.3390/ijms23126808
Sato, C., Morgan, A., Lang, A., Salehi-Rad, S., Kawarai, T., Meng, Y., … & Rogaeva, E. (2005). Analysis of the glucocerebrosidase gene in parkinson’s disease. Movement Disorders, 20(3), 367-370. https://doi.org/10.1002/mds.20319
Sawant, V., Park, H., Baek, S., Lee, J., Choi, J., Park, K., … & Choo, H. (2019). Benzoxazoles as selective monoamine oxidase b (mao‐b) inhibitors. Bulletin of the Korean Chemical Society, 40(5), 457-460. https://doi.org/10.1002/bkcs.11704
Shook, B. and Jackson, P. (2011). Adenosine a2a receptor antagonists and parkinson’s disease. Acs Chemical Neuroscience, 2(10), 555-567. https://doi.org/10.1021/cn2000537
Shulman, L., Wen, X., Weiner, W., Bateman, D., Minagar, A., Duncan, R., … & Konefal, J. (2002). Acupuncture therapy for the symptoms of parkinson’s disease. Movement Disorders, 17(4), 799-802. https://doi.org/10.1002/mds.10134
Southwell, D., Nicholas, C., Basbaum, A., Stryker, M., Kriegstein, A., Rubenstein, J., … & Alvarez-Buylla, A. (2014). Interneurons from embryonic development to cell-based therapy. Science, 344(6180). https://doi.org/10.1126/science.1240622
Stahl, S. (2016). Parkinson’s disease psychosis as a serotonin-dopamine imbalance syndrome. CNS Spectrums, 21(5), 355-359. https://doi.org/10.1017/s1092852916000602
Tanabe, A., Yamamura, Y., Kasahara, J., Morigaki, R., Kaji, R., & Goto, S. (2014). A novel tyrosine kinase inhibitor amn107 (nilotinib) normalizes striatal motor behaviors in a mouse model of parkinson’s disease. Frontiers in Cellular Neuroscience, 8. https://doi.org/10.3389/fncel.2014.00050
Tang, S., Wang, A., Yan, X., Chu, L., Yang, X., Song, Y., … & Xue, P. (2019). Brain-targeted intranasal delivery of dopamine with borneol and lactoferrin co-modified nanoparticles for treating parkinson’s disease. Drug Delivery, 26(1), 700-707. https://doi.org/10.1080/10717544.2019.1636420
Zhang, S., Zou, Z., Jiang, X., Xu, R., Zhang, W., Zhou, Y., … & Ke, Y. (2007). The therapeutic effects of tyrosine hydroxylase gene transfected hematopoetic stem cells in a rat model of parkinson’s disease. Cellular and Molecular Neurobiology, 28(4), 529-543. https://doi.org/10.1007/s10571-007-9191-8
Zhang, X., Feng, Z., & Chergui, K. (2014). Allosteric modulation of glun2c/glun2d‐containing nmda receptors bidirectionally modulates dopamine release: implication for parkinson’s disease. British Journal of Pharmacology, 171(16), 3938-3945. https://doi.org/10.1111/bph.12758