Nanoparticles: Synthesis, Characterization, and Multidisciplinary Applications
This review investigates nanoparticles, focusing on their synthesis, characterization, and wide-ranging applications. Nanoparticles provide novel solutions in fields ranging from medicine to materials science, highlighting their importance in advancing technology and enhancing human health.
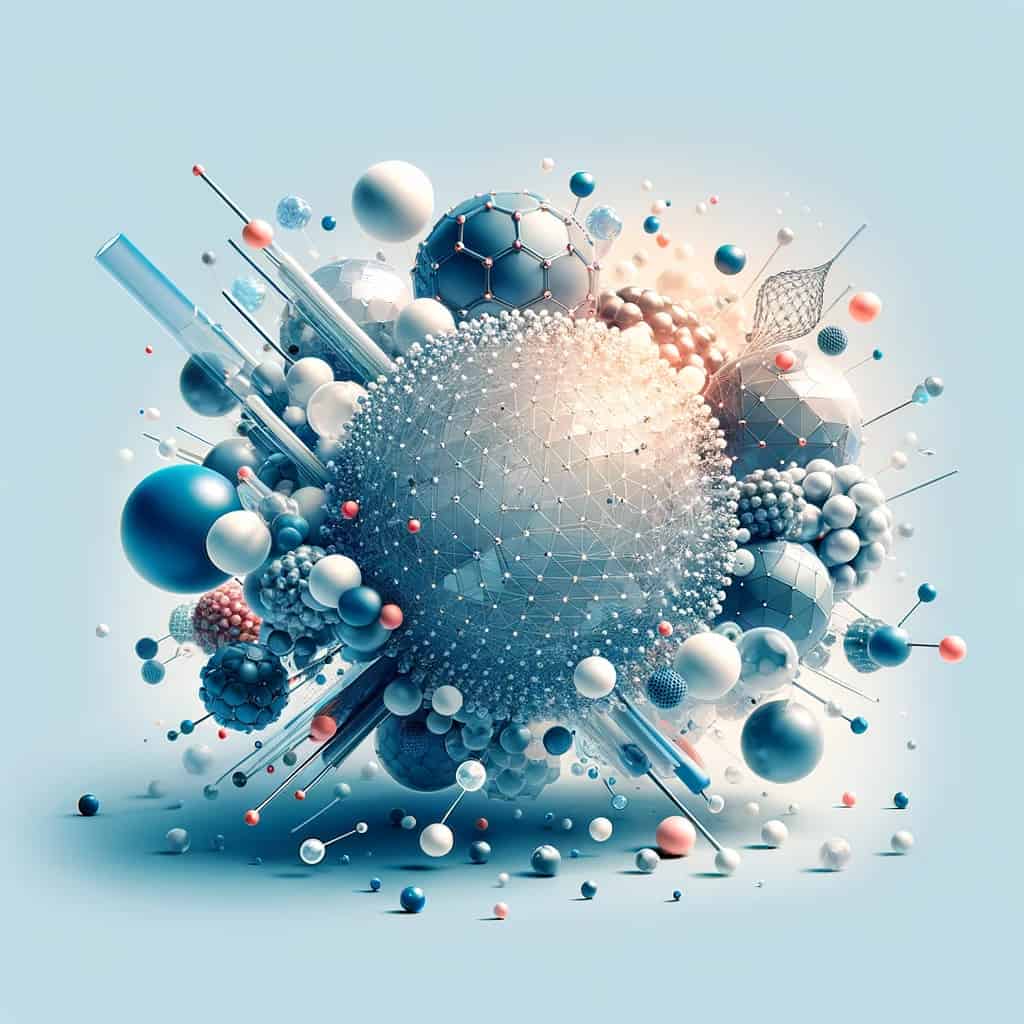
Introduction to Nanoparticles
Nanoparticles are materials with at least one dimension less than 100 nanometers (Aleksandrowicz-Trzcińska et al., 2018). These nanoscale dimensions result in unique features that differ from both bulk materials and individual molecules (Nune et al., 2009). Nanoparticles’ optical, electrical, and magnetic properties vary with size and shape (Choueiri et al., 2016). When nanoparticles are placed in multidimensional arrays, their collective properties can be modified by varying the array dimensions, interparticle spacing, and array form (Ross et al., 2016).
Nanoparticles play a significant role in scientific research, with applications ranging from health to electronics to materials science. Nanoparticles have showed potential in improving endodontic infection treatments due to their increased antibacterial activity and reactivity (Wong et al., 2021). Nanoparticles have also been used in biological imaging because they have unique features that distinguish them from molecules and bulk materials (Nune et al., 2009). Nanoparticles have been used in materials science for electronic, optical, and sensor applications, taking advantage of their interesting features due to their nanoscale dimensions (Shipway et al., 2000).
Furthermore, the size of nanoparticles influence their properties and uses. For example, varying the size of chiral gold nanoparticles can result in changes in helical pitch and nanoparticle diameters, allowing for the assembly of a variety of shapes (Mokashi-Punekar et al., 2017). Similarly, the size and surface effects of nanoparticles, such as Prussian Blue nanoparticles, can be altered through interactions with organic polymers (Uemura et al., 2004). Furthermore, controlled synthesis of nanoparticles, such as magnetic iron oxide nanoparticles, entails studying the size of nanoparticles under various synthesis circumstances in order to adjust their physical and chemical properties (Schwaminger et al., 2020).
Nanoparticles, defined by their nanoscale dimensions, have unique features that make them useful in a variety of scientific disciplines. Their size-dependent optical, electrical, and magnetic capabilities have enabled advances in medicine, electronics, and materials science, demonstrating nanoparticles’ diversity and importance in modern research and applications.
Synthesis and Characterization of Nanoparticles
Chemical reduction, sol-gel techniques, and biological synthesis are some of the ways used to create nanoparticles. Chemical reduction reduces metal ions to make nanoparticles, whereas sol-gel methods produce nanoparticles by hydrolyzing and condensing metal alkoxides. Biological synthesis is the utilization of biological entities such as plants or microbes to produce nanoparticles (Mourdikoudis et al., 2018; Kouhpanji and Stadler, 2020; Ahmed & Mustafa, 2019).
Characterization procedures are critical in determining the size, shape, and chemical content of nanoparticles. Transmission Electron Microscopy (TEM) produces high-resolution images of nanoparticles, allowing for the observation of their shape. Nanoparticle surface morphology is studied using Scanning Electron Microscopy (SEM). X-ray diffraction (XRD) is used to determine the crystalline structure and phase composition of nanoparticles (Mourdikoudis et al., 2018; Kouhpanji and Stadler, 2020; Petrushevska et al., 2019).
TEM and SEM are useful for visualizing the size and shape of nanoparticles, whereas XRD is required to determine the crystal structure of nanoparticles. These methodologies complement one another, resulting in a full understanding of nanoparticle properties. Furthermore, Dynamic Light Scattering (DLS) can determine the size distribution of nanoparticles in solution, whereas Mass Spectrometry can analyze the elemental composition of nanoparticles (Mourdikoudis et al., 2018; Kouhpanji and Stadler, 2020; Petrushevska et al., 2019).
Nanoparticles must be synthesized using various methods and characterized using techniques such as TEM, SEM, XRD, DLS, and Mass Spectrometry in order to understand and optimize their properties for a wide range of applications in nanotechnology, medicine, and materials science.
Applications of Nanoparticles
Nanoparticles have showed tremendous promise in a variety of sectors, proving their ability to address difficult problems. Nanoparticles are important in medicine because they improve drug delivery methods and therapy efficacy. For example, silver nanoparticles have been shown to be beneficial in treating antibiotic-resistant bacteria in wastewater (Anele et al., 2022). Similarly, iron oxide nanoparticles have been effectively employed in soil and groundwater remediation to help remove contaminants such as heavy metals (Galdames et al., 2020). These applications demonstrate the adaptability of nanoparticles in addressing environmental issues.
In engineering, nanoparticles have played an important role in improving material characteristics. Copper oxide nanoparticles have been used in a variety of industrial applications, including catalysis and environmental remediation (Sun et al., 2012). Furthermore, zero-valent iron nanoparticles have been shown to be successful in treating soil and groundwater contaminated with organic compounds, demonstrating their utility in environmental remediation (Chidambaram et al., 2010). These research demonstrate the importance of nanoparticles in improving material characteristics for engineering applications.
Nanoparticles have also played an important role in environmental cleanup, providing long-term solutions to pollution issues. Magnetic nanoparticles, notably maghemite nanoparticles, have proved critical in water purification and environmental restoration (Tang & Lo, 2013). Furthermore, stabilized nanoparticles have demonstrated promise in the in-situ remediation of metal-contaminated soil and groundwater, demonstrating their potential for long-term environmental effect (Liu et al., 2015). These studies highlight nanoparticles’ numerous potential in combating environmental degradation and fostering sustainability.
To summarize, nanoparticles have emerged as strong instruments with numerous applications in medicine, engineering, and environmental cleanup. Key studies concentrating on medicine delivery systems, material property enhancement, and environmental remediation demonstrate nanoparticles’ potential to address complicated challenges and contribute to scientific progress.
References:
Ahmed, R. and Mustafa, D. (2019). Green synthesis of silver nanoparticles mediated by traditionally used medicinal plants in sudan. International Nano Letters, 10(1), 1-14. https://doi.org/10.1007/s40089-019-00291-9
Aleksandrowicz-Trzcińska, M., Olchowik, J., & Drozdowski, S. (2018). Effects of copper and silver nanoparticles on growth of selected species of pathogenic and wood-decay fungi in vitro. The Forestry Chronicle, 94(02), 109-116. https://doi.org/10.5558/tfc2018-017
Anele, A., Obare, S., & Wei, J. (2022). Recent trends and advances of co3o4 nanoparticles in environmental remediation of bacteria in wastewater. Nanomaterials, 12(7), 1129. https://doi.org/10.3390/nano12071129
Chidambaram, D., Hennebel, T., Taghavi, S., Mast, J., Boon, N., Verstraete, W., … & Fitts, J. (2010). Concomitant microbial generation of palladium nanoparticles and hydrogen to immobilize chromate. Environmental Science & Technology, 44(19), 7635-7640. https://doi.org/10.1021/es101559r
Choueiri, R., Galati, E., Thérien-Aubin, H., Klinkova, A., Larin, E., Querejeta-Fernández, A., … & Kumacheva, E. (2016). Surface patterning of nanoparticles with polymer patches. Nature, 538(7623), 79-83. https://doi.org/10.1038/nature19089
Galdames, A., Ruiz‐Rubio, L., Orueta, M., Sánchez-Arzalluz, M., & Vilas, J. (2020). Zero-valent iron nanoparticles for soil and groundwater remediation. International Journal of Environmental Research and Public Health, 17(16), 5817. https://doi.org/10.3390/ijerph17165817
Kouhpanji, M. and Stadler, B. (2020). A guideline for effectively synthesizing and characterizing magnetic nanoparticles for advancing nanobiotechnology: a review. Sensors, 20(9), 2554. https://doi.org/10.3390/s20092554
Liu, W., Tian, S., Zhao, X., Xie, W., & Gong, Y. (2015). Application of stabilized nanoparticles for in situ remediation of metal-contaminated soil and groundwater: a critical review. Current Pollution Reports, 1(4), 280-291. https://doi.org/10.1007/s40726-015-0017-x
Mokashi-Punekar, S., Merg, A., & Rosi, N. (2017). Systematic adjustment of pitch and particle dimensions within a family of chiral plasmonic gold nanoparticle single helices. Journal of the American Chemical Society, 139(42), 15043-15048. https://doi.org/10.1021/jacs.7b07143
Mourdikoudis, S., Pallares, R., & Thanh, N. (2018). Characterization techniques for nanoparticles: comparison and complementarity upon studying nanoparticle properties. Nanoscale, 10(27), 12871-12934. https://doi.org/10.1039/c8nr02278j
Nune, S., Gunda, P., Thallapally, P., Lin, Y., Forrest, M., & Berkland, C. (2009). Nanoparticles for biomedical imaging. Expert Opinion on Drug Delivery, 6(11), 1175-1194. https://doi.org/10.1517/17425240903229031
Petrushevska, M., Pavlovska, K., Laskova, J., Zdravkovski, P., & Dodov, M. (2019). Transmission electron microscopy: novel application of established technique in characterization of nanoparticles as drug delivery systems. Prilozi, 40(2), 67-72. https://doi.org/10.2478/prilozi-2019-0016
Ross, M., Mirkin, C., & Schatz, G. (2016). Optical properties of one-, two-, and three-dimensional arrays of plasmonic nanostructures. The Journal of Physical Chemistry C, 120(2), 816-830. https://doi.org/10.1021/acs.jpcc.5b10800
Schwaminger, S., Syhr, C., & Berensmeier, S. (2020). Controlled synthesis of magnetic iron oxide nanoparticles: magnetite or maghemite?. Crystals, 10(3), 214. https://doi.org/10.3390/cryst10030214
Shipway, A., Katz, E., & Willner, I. (2000). Nanoparticle arrays on surfaces for electronic, optical, and sensor applications. Chemphyschem, 1(1), 18-52. https://doi.org/10.1002/1439-7641(20000804)1:13.0.co;2-l
Sun, T., Yan, Y., Zhao, Y., Guo, F., & Jiang, C. (2012). Copper oxide nanoparticles induce autophagic cell death in a549 cells. Plos One, 7(8), e43442. https://doi.org/10.1371/journal.pone.0043442
Tang, S. and Lo, I. (2013). Magnetic nanoparticles: essential factors for sustainable environmental applications. Water Research, 47(8), 2613-2632. https://doi.org/10.1016/j.watres.2013.02.039
Uemura, T., Ohba, M., & Kitagawa, S. (2004). Size and surface effects of prussian blue nanoparticles protected by organic polymers. Inorganic Chemistry, 43(23), 7339-7345. https://doi.org/10.1021/ic0488435
Wong, J., Zou, T., Lee, A., & Zhang, C. (2021). The potential translational applications of nanoparticles in endodontics. International Journal of Nanomedicine, Volume 16, 2087-2106. https://doi.org/10.2147/ijn.s293518