Membrane Lipids: Synthesis to Therapeutic Insights
This study examines the crucial function of membrane lipids in the organization of cells, the transmission of signals, and the processes of diseases. It emphasizes current progress in the production of lipids and potential approaches to treat lipid metabolism.
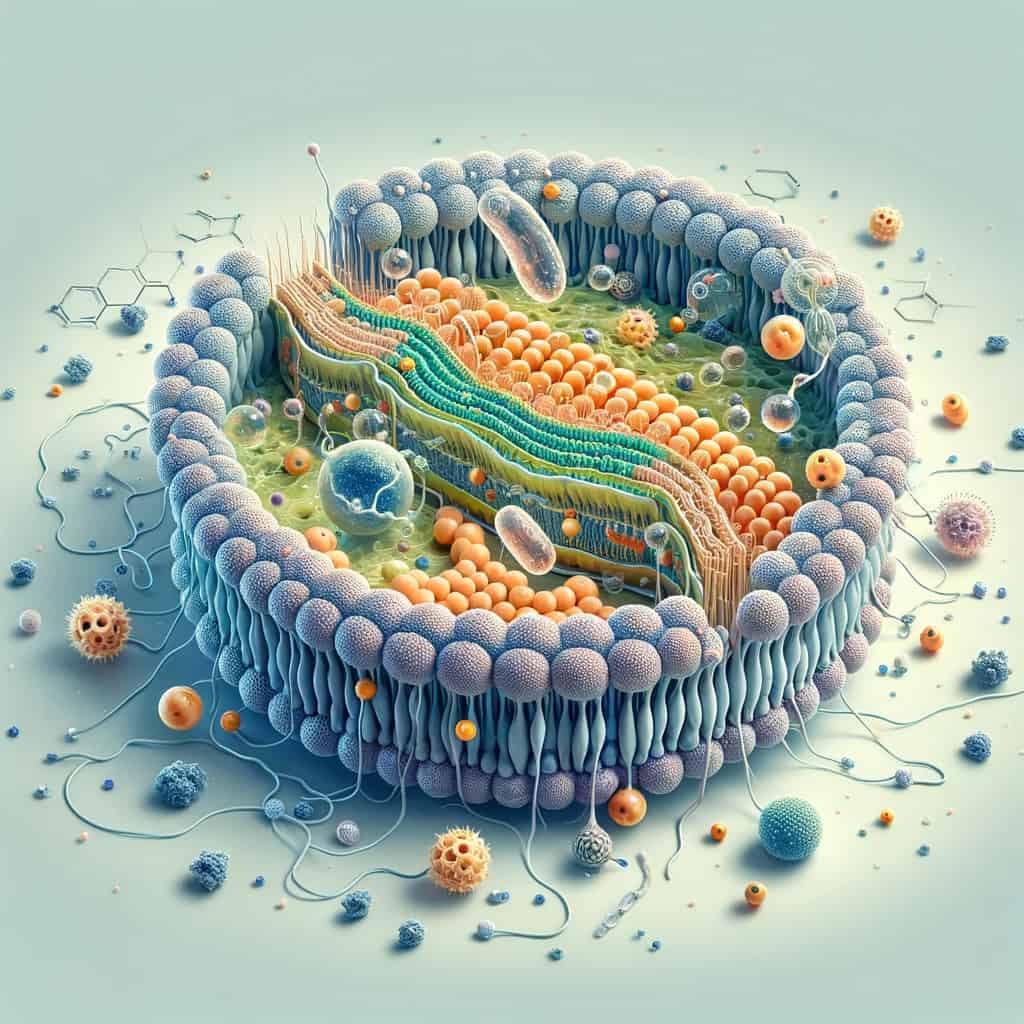
Introduction to Membrane Lipids
Cellular membranes rely on membrane lipids as crucial constituents, playing a vital role in maintaining their structural integrity and fluidity. The maintenance of cell function and dynamics relies heavily on the presence of lipids, including phospholipids, glycolipids, and sterols (Zhang et al., 2011). The synthesis of membrane lipids is a complex process that is subject to strict regulation in order to maintain lipid equilibrium and cellular stability (Xu et al., 2008). The perturbation of lipid production pathways has the potential to influence the dynamics of membranes and the functioning of cells (Liu et al., 2012).
Moreover, membrane lipids have the dual purpose of offering structural reinforcement and exerting a substantial influence on cellular communication and pathological mechanisms. Zhang et al. (2011) have identified glycosphingolipids as crucial factors in determining cell polarity and membrane structure. The regulation of lipid biosynthesis in liver cells is mediated by the membrane protein gp78, which plays a crucial role in enabling the degradation of essential enzymes involved in lipid formation. This process has implications for the development of hyperlipidemia and insulin resistance (Liu et al., 2012). Moreover, the involvement of membrane lipids in protein secretion processes highlights their multifaceted functions within cellular processes (Melser et al., 2010).
Numerous studies have provided evidence regarding the crucial significance of membrane lipid production in diverse biological mechanisms. Active glycolysis and fatty acid production play a crucial role in the growth of nodules in plant root nodulation (Zhang et al., 2020). Furthermore, the lipid manufacturing pathway plays a vital role in the pathogenicity and viability of bacteria, highlighting the importance of membrane lipids in the development of microbial diseases (Touchette et al., 2017). This route is a central focus in cancer research, emphasizing its significance in the advancement of the disease and prospective approaches for treatment (Xu et al., 2022).
Membrane lipid production involves intricate enzymatic processes and interactions. Glycerol-3-phosphate acyltransferases, which are proteins, play a crucial role in the process of lipid biosynthesis in plants. They contribute to the creation of glycerolipids, which are necessary for the structure of cell membranes (Chen et al., 2011). Moreover, the lipid production process in archaea encompasses a distinctive isoprenoid biochemistry, resulting in the creation of specific membrane lipid structures (Lai et al., 2009).
Mesoporous lipids are essential constituents of cellular membranes, serving a wide range of tasks including providing structural support, facilitating cell signaling, and participating in pathological processes. Comprehending the process of creating and controlling membrane lipids is essential for determining their functions in cellular function and disease.
Biosynthesis and Regulation
In order to gain a comprehensive understanding of the biosynthesis and regulation of significant membrane lipids, it is imperative to investigate the pathways implicated in lipid production as well as the regulatory mechanisms that maintain lipid equilibrium and systemic stability within the cellular environment. A multitude of research have provided insights into these complex processes and their impact on the dynamics and functionality of membranes.
Hagen et al. (2010) conducted a study that highlights the significance of the sterol regulatory element binding protein 1 (SREBP1) in the regulation of desaturase enzymes, hence exerting control over the manufacture of unsaturated fatty acids. This observation underscores the complex regulatory networks that govern pathways involved in lipid production. In addition, Morita et al. (2005) offer valuable information on the segregation of enzymes responsible for the production of vital lipid categories in the plasma membrane, highlighting the important spatial arrangement necessary for lipid metabolism.
Moreover, the research conducted by Xu et al. (2008) about the movement of lipids between the endoplasmic reticulum and plastids in Arabidopsis provides a clear understanding of the processes involved in lipid transfer, which is crucial for preserving the composition of membrane lipids. This study highlights the importance of lipid transport mechanisms in maintaining appropriate equilibrium and functionality of membrane lipids.
Furthermore, the study conducted by Sharma et al. (2010) about the inhibition of sterol biosynthesis and its subsequent effect on tombusvirus replication underscores the crucial involvement of lipids in viral mechanisms, hence illustrating the wider ramifications of lipid biosynthesis beyond cellular activities. Furthermore, the research conducted by Yang et al. (2021) regarding the buildup of lipids in oleaginous fungus resulting from the removal of plasma membrane malate transporters elucidates the complex interplay between lipid biosynthesis and the regulation of cellular lipid content.
This collection of papers collectively underscores the complex regulatory mechanisms and pathways implicated in the manufacture of membrane lipids, underscoring the significance of preserving lipid equilibrium for cellular homeostasis and optimal membrane functionality.
Role in Cell Signaling and Disease
Recent studies have investigated the complex interplay between membrane lipids and their influence on cellular signaling pathways and the progression of diseases. The significance of lipid abnormalities in a range of diseases, including neurodegenerative disorders and cardiovascular diseases, has been underscored by numerous studies, underscoring the possibility for therapeutic approaches.
As an illustration, Ha et al. (2014) undertook a comprehensive examination and statistical analysis to evaluate the influence of dietary pulse consumption on well-established therapeutic lipid targets aimed at mitigating cardiovascular risk. Insights into the possibility of dietary treatments to alter lipid profiles and lower cardiovascular risks are provided by this study. Florens et al. (2016) conducted a study that examined the alterations of lipoproteins in individuals with chronic renal illness and their potential consequences for cardiovascular disorders. The researchers placed particular emphasis on the role of modified lipids as uremic toxins.
In addition, He et al. (2018) conducted a study to investigate the impact of low-level lead exposure on cardiovascular disease. The researchers specifically focused on the involvement of telomere shortening and lipid disruption in mediating the cardiovascular consequences of environmental exposure. the study conducted by Kuliszkiewicz-Janus et al. In their study, KuliszkiewiczJanus et al. (2008) examined the changes in lipid profiles in hematologic cancers, providing valuable information about the changes in lipid profiles that are linked to disease activity in these diseases.
Furthermore, a comprehensive review and meta-analysis was undertaken by Hsu et al. (2020) to assess the effectiveness of more intensive lipid-lowering therapy in the treatment of cardiovascular disorders. This study highlights the significance of lipid management in mitigating cardiovascular risks. The preventative effects of catechins on cardiovascular disease were examined by Chen et al. (2016), with a particular focus on the possible advantages of catechins in regulating lipid metabolism and promoting cardiovascular well-being.
In summary, recent studies have yielded significant findings about the intricate functions of membrane lipids in cellular signaling cascades and pathological mechanisms. The comprehension of the influence of lipid abnormalities on the progression of diseases, specifically in the domains of neurodegenerative and cardiovascular disorders, presents prospects for focused therapeutic interventions with the objective of enhancing health outcomes.
References:
Chen, X., Hu, T., Han, Y., Huang, W., Yuan, H., Zhang, Y., … & Jiang, Y. (2016). Preventive effects of catechins on cardiovascular disease. Molecules, 21(12), 1759. https://doi.org/10.3390/molecules21121759
Chen, X., Snyder, C., Truksa, M., Shah, S., & Weselake, R. (2011). sn-glycerol-3-phosphate acyltransferases in plants. Plant Signaling & Behavior, 6(11), 1695-1699. https://doi.org/10.4161/psb.6.11.17777
Florens, N., Calzada, C., Lyasko, E., Juillard, L., & Soulage, C. (2016). Modified lipids and lipoproteins in chronic kidney disease: a new class of uremic toxins. Toxins, 8(12), 376. https://doi.org/10.3390/toxins8120376
Ha, V., Sievenpiper, J., Souza, R., Jayalath, V., Mirrahimi, A., Agarwal, A., … & Jenkins, D. (2014). Effect of dietary pulse intake on established therapeutic lipid targets for cardiovascular risk reduction: a systematic review and meta-analysis of randomized controlled trials. Canadian Medical Association Journal, 186(8), E252-E262. https://doi.org/10.1503/cmaj.131727
Hagen, R., Rodríguez‐Cuenca, S., & Vidal-Puig, A. (2010). An allostatic control of membrane lipid composition by srebp1. Febs Letters, 584(12), 2689-2698. https://doi.org/10.1016/j.febslet.2010.04.004
He, L., Chen, Z., Dai, B., Li, G., & Zhu, G. (2018). Low-level lead exposure and cardiovascular disease: the roles of telomere shortening and lipid disturbance. The Journal of Toxicological Sciences, 43(11), 623-630. https://doi.org/10.2131/jts.43.623
Hsu, H., Lin, C., Lee, Y., Wu, T., & Chien, K. (2020). Efficacy of more intensive lipid-lowering therapy on cardiovascular diseases: a systematic review and meta-analysis. BMC Cardiovascular Disorders, 20(1). https://doi.org/10.1186/s12872-020-01567-1
Kuliszkiewicz‐Janus, M., Małecki, R., & Mohamed, A. (2008). Lipid changes occuring in the course of hematological cancers. Cellular & Molecular Biology Letters, 13(3). https://doi.org/10.2478/s11658-008-0014-9
Lai, D., Lluncor, B., Schröder, I., Gunsalus, R., Liao, J., & Monbouquette, H. (2009). Reconstruction of the archaeal isoprenoid ether lipid biosynthesis pathway in escherichia coli through digeranylgeranylglyceryl phosphate. Metabolic Engineering, 11(3), 184-191. https://doi.org/10.1016/j.ymben.2009.01.008
Liu, T., Tang, J., Li, P., Shen, Y., Li, J., Miao, H., … & Song, B. (2012). Ablation of gp78 in liver improves hyperlipidemia and insulin resistance by inhibiting srebp to decrease lipid biosynthesis. Cell Metabolism, 16(2), 213-225. https://doi.org/10.1016/j.cmet.2012.06.014
Melser, S., Batailler, B., Peypelut, M., Poujol, C., Bellec, Y., Wattelet-Boyer, V., … & Moreau, P. (2010). Glucosylceramide biosynthesis is involved in golgi morphology and protein secretion in plant cells. Traffic, 11(4), 479-490. https://doi.org/10.1111/j.1600-0854.2009.01030.x
Morita, Y., Velasquez, R., Taig, E., Waller, R., Patterson, J., Tull, D., … & McConville, M. (2005). Compartmentalization of lipid biosynthesis in mycobacteria. Journal of Biological Chemistry, 280(22), 21645-21652. https://doi.org/10.1074/jbc.m414181200
Sharma, M., Sasvari, Z., & Nagy, P. (2010). Inhibition of sterol biosynthesis reduces tombusvirus replication in yeast and plants. Journal of Virology, 84(5), 2270-2281. https://doi.org/10.1128/jvi.02003-09
Touchette, M., Vlack, E., Bai, L., Kim, J., Cognetta, A., Previti, M., … & Seeliger, J. (2017). A screen for protein–protein interactions in live mycobacteria reveals a functional link between the virulence-associated lipid transporter lprg and the mycolyltransferase antigen 85a. Acs Infectious Diseases, 3(5), 336-348. https://doi.org/10.1021/acsinfecdis.6b00179
Xu, C., Fan, J., Cornish, A., & Benning, C. (2008). Lipid trafficking between the endoplasmic reticulum and the plastid in arabidopsis requires the extraplastidic tgd4 protein. The Plant Cell, 20(8), 2190-2204. https://doi.org/10.1105/tpc.108.061176
Xu, Y., Jin, Y., Gao, S., Wang, Y., Qu, C., Wu, Y., … & Liu, S. (2022). Prognostic signature and therapeutic value based on membrane lipid biosynthesis-related genes in breast cancer. Journal of Oncology, 2022, 1-16. https://doi.org/10.1155/2022/7204415
Yang, J., Cánovas-Márquez, J., Li, P., Li, S., Niu, J., Wang, X., … & Song, Y. (2021). Deletion of plasma membrane malate transporters increased lipid accumulation in the oleaginous fungus mucor circinelloides wj11. Journal of Agricultural and Food Chemistry, 69(33), 9632-9641. https://doi.org/10.1021/acs.jafc.1c03307
Zhang, G., Ahmad, M., Chen, B., Manan, S., Zhang, Y., Jin, H., … & Zhao, J. (2020). Lipidomic and transcriptomic profiling of developing nodules reveals the essential roles of active glycolysis and fatty acid and membrane lipid biosynthesis in soybean nodulation. The Plant Journal, 103(4), 1351-1371. https://doi.org/10.1111/tpj.14805
Zhang, H., Abraham, N., Khan, L., Hall, D., Fleming, J., & Gobel, V. (2011). Apicobasal domain identities of expanding tubular membranes depend on glycosphingolipid biosynthesis. Nature Cell Biology, 13(10), 1189-1201. https://doi.org/10.1038/ncb2328