Liquid Methane: the Fuel of SpaceX Starship
NASA officials celebrated SpaceX’s third Starship test flight on March 14, 2024, which marked significant progress toward enabling astronauts to land on the moon. The flight, which launched from SpaceX’s Starbase in South Texas, achieved orbital velocity and successfully demonstrated propellant transfer in space, a critical capability for future NASA Artemis missions. Despite these milestones, it is acknowledged that extensive data review and additional work are required to improve the spacecraft’s performance. The test is consistent with SpaceX’s goal of achieving ambitious goals with each flight to improve future mission capabilities, particularly the Human Landing System (HLS) for Artemis 3, which is scheduled for no earlier than September 2026. Vehicle roll rates during the coast phase were identified as a challenge, and upcoming test flights will allow for rapid iteration on the vehicle’s design and capabilities. NASA emphasizes the importance of repeated successes before committing to crewed missions, amidst ongoing development and technical evaluations of the Starship as a critical component of America’s lunar exploration strategy, in addition to contributions from other vendors such as Blue Origin.
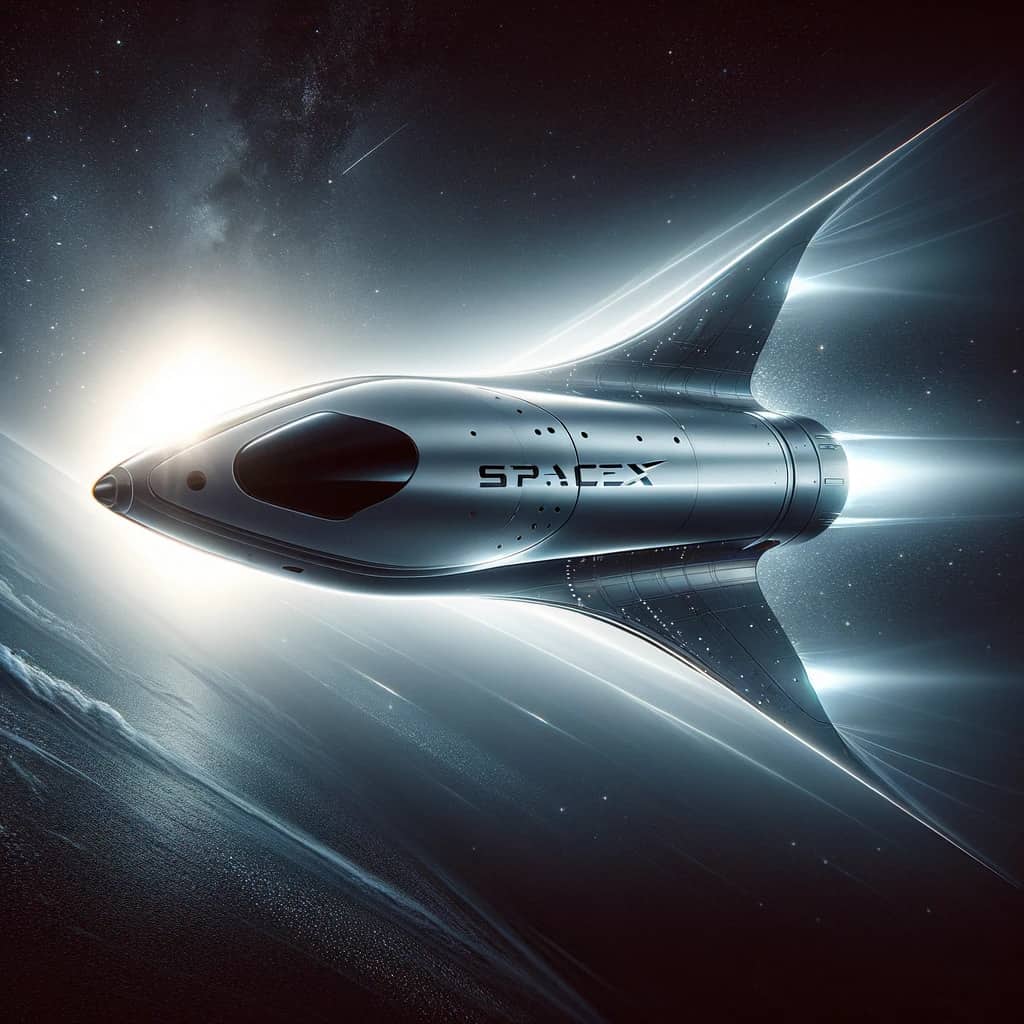
What’s the fuel of SpaceX Starship?
SpaceX’s Starship runs on a combination of liquid methane (CH4) and liquid oxygen (LOX). This decision is important for various reasons, including both engineering considerations and long-term strategic objectives:
Efficiency and Performance
High Specific Impulse: Methane and liquid oxygen have a high specific impulse, a measure of propulsion efficiency. This means that the Starship can generate greater thrust per unit of propellant, making it more efficient at transporting hefty payloads to orbit and beyond.
Lower Temperature: Methane may be stored at lower temperatures than hydrogen (another frequent rocket fuel when combined with oxygen). This enables more effective storage and less insulation, lowering the rocket’s weight.
Reusability & Sustainability
Clean Burning: Methane burns cleanly with oxygen, emitting largely carbon dioxide and water. This results in fewer soot deposits in the engines, which is beneficial to the reusability of the Starship engines by shortening maintenance and refurbishment durations between flights.
Resource Availability on Mars: SpaceX’s long-term goal includes flights to Mars, where methane may be manufactured from the Martian atmosphere (which is high in carbon dioxide) and subsurface ice (which contains hydrogen). This mechanism, known as the Sabatier reaction, would produce methane and water, making Starship a more sustainable choice for interplanetary travel by allowing ships to be refueled on Mars.
Economic and practical considerations
Cost-Effective: In comparison to other propellants such as hydrogen, methane is relatively affordable and abundantly available, which can assist cut the costs of space missions.
Ease of Handling: Methane is easier to handle and store than hydrogen, which requires extremely low temperatures to remain liquid and has a greater leak rate due to its small molecular size.
In summary, SpaceX chose methane and liquid oxygen as the fuel for its Starship based on efficiency, performance, and the strategic goal of supporting long-term human presence on Mars, as well as practical and economic benefits.
The advances in liquid methane
Liquid methane, a fundamental component of natural gas, has been intensively researched due to its usefulness in a variety of applications, including energy storage, gas transportation, and climate implications. Research has been conducted to better understand the behavior of liquid methane in various settings. For example, research have focused on the structural features of liquid methane and methane/water mixtures, emphasizing the significance of correct representations of short-range interactions in order to quantitatively describe solvation structures (Riera et al., 2020). Furthermore, the interaction of water and methane hydrate crystals has been studied for its implications in flow assurance and renewable energy resources (Mirzaeifard et al., 2019).
The adsorption of methane in mesoporous silica materials has been investigated, highlighting the effect of pore size on methane adsorption due to the rough surface of the material (Chiang et al., 2016). Furthermore, the high harmonic production in liquids such as methane has been investigated to better understand the impact of the liquid’s chemical characteristics on spectra (Neufeld et al., 2022). Furthermore, the hydrophobic interaction between methane and graphene in aqueous solutions has been described using ab initio simulations, providing insight into methane’s solvation properties (Calero et al., 2013).
Studies have also investigated the phase equilibria of methane mixtures, such as methane + decane systems, in order to better understand vapor-liquid equilibria and physical properties at various temperatures (Kariznovi et al., 2012). Furthermore, the phase behavior of hydrocarbons in nanopores, including methane, has been studied to provide insight into the thermodynamic behavior of confined fluids (Sobecki et al., 2019).
Overall, research on liquid methane is conducted across multiple fields, including chemistry, physics, and materials science, with the goal of improving our understanding of its properties and behavior in diverse situations and mixes.
References:
Calero, C., Martí, J., Guàrdia, E., & Masia, M. (2013). Characterization of the methane–graphene hydrophobic interaction in aqueous solution from ab initio simulations. Journal of Chemical Theory and Computation, 9(11), 5070-5075. https://doi.org/10.1021/ct400554q
Chiang, W., Fratini, E., Chen, J., & Liu, Y. (2016). Pore size effect on methane adsorption in mesoporous silica materials studied by small-angle neutron scattering. Langmuir, 32(35), 8849-8857. https://doi.org/10.1021/acs.langmuir.6b02291
Kariznovi, M., Nourozieh, H., & Abedi, J. (2012). Vapor–liquid phase equilibria and physical properties measurements for ternary systems (methane + decane + hexadecane). Journal of Chemical & Engineering Data, 57(9), 2535-2542. https://doi.org/10.1021/je300482b
Mirzaeifard, S., Servio, P., & Rey, A. (2019). Multiscale modeling and simulation of water and methane hydrate crystal interface. Crystal Growth & Design, 19(9), 5142-5151. https://doi.org/10.1021/acs.cgd.9b00578
Neufeld, O., Nourbakhsh, Z., Tancogne-Dejean, N., & Rubio, Á. (2022). Ab initio cluster approach for high harmonic generation in liquids. Journal of Chemical Theory and Computation, 18(7), 4117-4126. https://doi.org/10.1021/acs.jctc.2c00235
Riera, M., Hirales, A., Ghosh, R., & Paesani, F. (2020). Data-driven many-body models with chemical accuracy for ch4/h2o mixtures. The Journal of Physical Chemistry B, 124(49), 11207-11221. https://doi.org/10.1021/acs.jpcb.0c08728
Sobecki, N., Nieto-Draghi, C., Lella, A., & Ding, D. (2019). Phase behavior of hydrocarbons in nano-pores. Fluid Phase Equilibria, 497, 104-121. https://doi.org/10.1016/j.fluid.2019.05.025