Horizons in Click & Bioorthogonal Chemistry for Biomedical Applications
This study examines the cutting-edge topics of click and bioorthogonal chemistry, both of which are critical for advances in biomedical research. It emphasizes the techniques’ selectivity, effectiveness, and compatibility with biological systems, highlighting their applications in biomaterials, drug development, and diagnostics. Despite their triumphs, concerns with reaction kinetics and biocompatibility demonstrate how much more work remains to be done. The future directions’ key goals are to improve reaction conditions, expand bioorthogonal toolkits, and investigate innovative biomedical applications. In addition to enhancing molecular targeting and diagnostics, this synthesis of click and bioorthogonal chemistry paves the way for next-generation therapeutics.
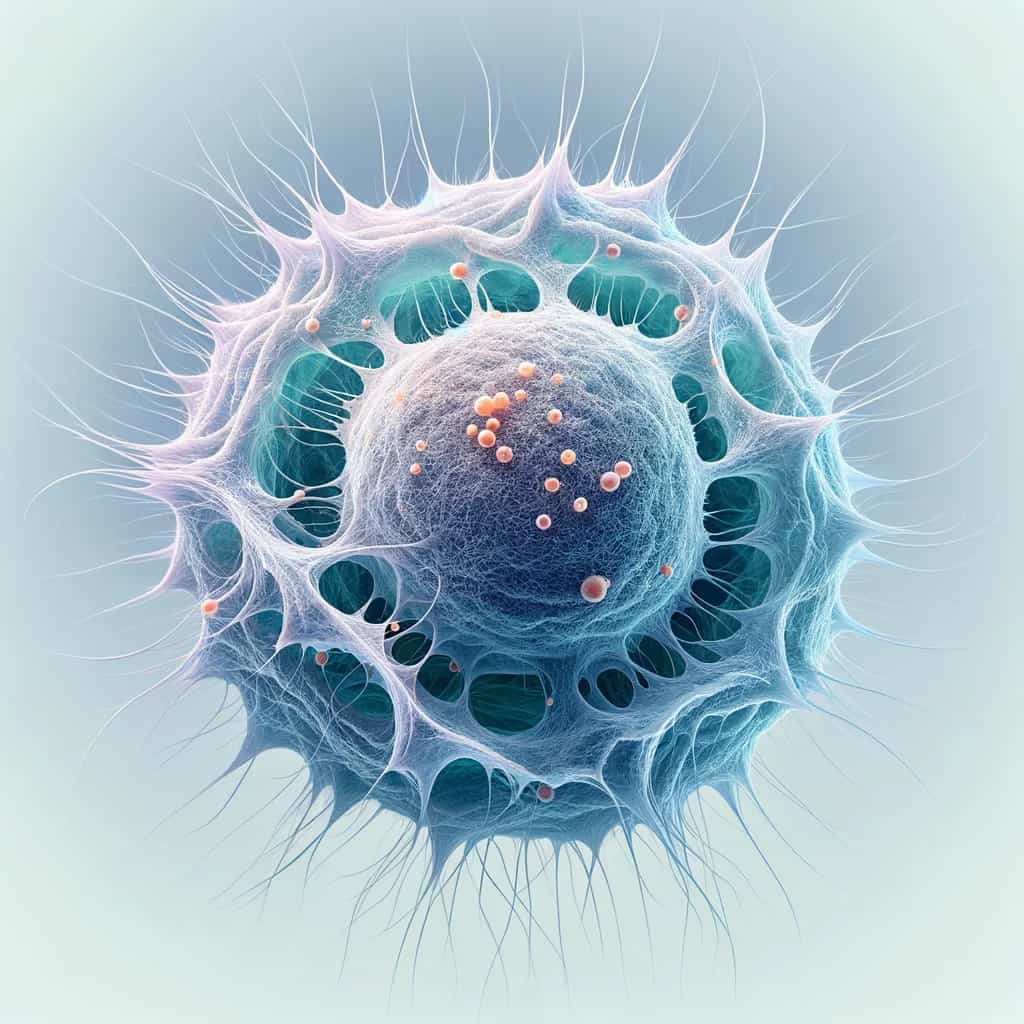
Introduction to Click & Bioorthogonal Chemistry
Click chemistry is known for its biocompatibility, efficiency, and selectivity (Li et al., 2013). According to Li et al. (2013), it allows for the joining of molecular fragments in mild conditions, promoting precise molecular design. Bioorthogonal click chemistry, a subset of click chemistry, is particularly promising for applications within biological systems since it reacts rapidly, selectively, and biocompatiblely without creating byproducts (Battigelli et al., 2022).
Click and bioorthogonal chemistry are important because they can be used to functionalize materials, simplify complex molecular synthesis, and advance fields such as tissue engineering, drug delivery, nanomedicine, and chemical proteomics (Taiariol et al., 2021; Zhong et al., 2023; Pookpanratana et al., 2014). Click chemistry has significantly facilitated the development of new combinatorial chemical processes, particularly the formation of carbon-heteroatom bonds, which are frequently used in chemical proteomics. Furthermore, bioorthogonal click chemistry has been shown to improve PET and SPECT radiochemistry, providing new opportunities for cell surface engineering and radiolabeling (Zhong et al., 2023).
Click chemistry has made it easier to develop multifunctional hydrogel materials in tissue engineering, which are essential for activities such as bioprinting and tissue regeneration (Janarthanan & Noh, 2018; Mueller et al., 2022). For tissue engineering projects to be successful, gelation and breakdown rates must be properly regulated with click chemistry (Mueller et al., 2022). Furthermore, the versatility of click chemistry in manufacturing functional materials for specific purposes has been established by its use in the production of bioactive materials for bone replacement (Liu et al., 2023).
Click chemistry’s versatility is demonstrated by its applications in surface modification, macromolecular engineering, and the production of sequence-defined macromolecules. Click chemistry is an important tool in molecular engineering, facilitating the synthesis of complex macromolecular structures (Nebhani & Barner-Kowollik, 2009; Sumerlin & Vogt, 2009; Holloway et al., 2019).
Overall, click and bioorthogonal chemistry are important tools for precise molecular engineering in both artificial and biological systems. They offer rapid, precise, and biocompatible reactions that allow for the design and creation of complex molecular structures with minimal to no detrimental side effects. These approaches are critical to modern molecular research and applications because they have advanced domains such as chemical proteomics, drug delivery, tissue engineering, and nanomedicine.
Key Developments and Applications
Bioorthogonal chemistry, particularly click chemistry, has had a significant impact on biomedical research by opening up new avenues for applications like as targeted therapy, in vivo imaging, and drug development. These methods give targeted and precise tools for molecular engineering in both artificial and biological systems (Devaraj, 2018; Völker et al., 2014; Patel et al., 2015).
Therapeutic chemicals can now be released with precision and control thanks to the development of novel drug delivery methods enabled by bioorthogonal chemistry (Patel et al., 2015; Xie et al., 2019; Shieh and Bertozzi, 2014). Researchers have developed molecular imaging agents for disease diagnosis and treatment by attaching functional groups to therapeutically relevant proteins using bioorthogonal methods (Devaraj, 2018; Xie et al., 2019). According to Xie et al. (2019) and Shieh & Bertozzi (2014), this technique has the potential to increase medicine targeting and delivery efficiency, resulting in more effective and personalized therapeutic interventions.
Bioorthogonal chemistry has considerably benefited targeted therapy with the development of bioorthogonal smart probes that allow for the monitoring of biomolecules as well as the targeted delivery of imaging or therapeutic chemicals (Tonga et al., 2015; Shieh and Bertozzi, 2014). These probes react with their targets in a way that allows them to precisely label and photograph specific biological molecules, allowing personalized therapeutic techniques to be developed (Tonga et al., 2015; Shieh & Bertozzi, 2014). The ability to focus and transport therapeutic molecules to specific parts of the body may lead to better treatment outcomes and fewer adverse effects (Tonga et al., 2015; Shieh & Bertozzi, 2014).
Bioorthogonal chemistry has significantly improved imaging methodologies for viewing dynamic processes within biological systems in the realm of in vivo imaging (Zhang et al., 2022; Chen et al., 2019). Researchers have been able to track biomolecules, view disease processes, and follow therapy responses with high precision and sensitivity by developing bioorthogonal systems that can be activated in vivo. These imaging modalities increase diagnostic and therapeutic procedures by providing detailed information on biological processes, disease etiology, and treatment efficacy (Zhang et al., 2022; Chen et al., 2019).
Overall, bioorthogonal chemistry, particularly click chemistry, has transformed biomedical research by enabling precise molecular engineering for targeted therapy, in vivo imaging, and drug development. These technologies have opened up new avenues for the creation of personalized therapeutic interventions, novel drug delivery systems, and enhanced imaging tools that enable the viewing of biological processes within living systems. Further advances in bioorthogonal chemistry are expected to alter biomedical research and pave the way for novel diagnostic and therapeutic approaches.
Challenges, Limitations, and Future Perspectives
Expanding application scopes, enhancing biocompatibility, and increasing reaction efficiency are some of the major challenges in bioorthogonal chemistry. Researchers are confronting these challenges head on in order to broaden the discipline and open up new potential for molecular engineering in artificial and biological systems.
The effectiveness of bioorthogonal processes is critical for their practical application in a wide range of fields. Maximizing reaction speeds, selectivity, and yields poses challenges for ensuring speedy and efficient conversions (Li & Chen, 2016; Pałasz, 2016). Scientists are exploring new reaction mechanisms and catalysts to enhance bioorthogonal processes for faster and more consistent molecular modifications (Li & Chen, 2016; Pałasz, 2016).
Another significant challenge in bioorthogonal chemistry is biocompatibility, which refers to how effectively reaction components interact with biological systems. To be used successfully in vivo, bioorthogonal reactions must be non-toxic and not interfere with natural biochemical processes (Battigelli et al., 2022; Amo et al., 2010). To reduce cytotoxicity and promote compatibility with biological environments, researchers are still working on developing biocompatible reaction conditions and components (Battigelli et al., 2022; Amo et al., 2010).
Bioorthogonal chemistry’s application ranges can be increased by researching new reaction types, substrates, and functional groups (Azagarsamy & Anseth, 2012; Row & Prescher, 2018). Scientists are investigating novel bioorthogonal reactions and expanding the toolbox of available reactions to satisfy specific research aims and enable novel applications in drug development, imaging, and tissue engineering (Azagarsamy & Anseth, 2012; Row & Prescher, 2018).
Some future directions in bioorthogonal chemistry research include the development of adaptable nanoreactors for bioorthogonal catalysis, the study of dissociative bioorthogonal reactions for drug carriers and molecular probes, and the development of photo-controllable reactions for spatiotemporal control of bio-targets (Li et al., 2020; Kumar, 2024; Tu et al., 2019). With the goal of advancing the field of bioorthogonal chemistry, these cutting-edge techniques will give new instruments and methodologies for precise molecular engineering in complex biological systems.
To summarize, future bioorthogonal chemistry research will aim to improve reaction efficiency, promote biocompatibility, and widen the range of applications. Researchers are addressing these difficulties and exploring new research routes to advance the field of bioorthogonal chemistry and enable precise molecular engineering with high selectivity and little interference in both live and synthetic contexts.
References:
Amo, D., Wang, W., Jiang, H., Besanceney, C., Yan, A., Levy, M., … & Wu, P. (2010). Biocompatible copper(i) catalysts for in vivo imaging of glycans. Journal of the American Chemical Society, 132(47), 16893-16899. https://doi.org/10.1021/ja106553e
Azagarsamy, M. and Anseth, K. (2012). Bioorthogonal click chemistry: an indispensable tool to create multifaceted cell culture scaffolds. Acs Macro Letters, 2(1), 5-9. https://doi.org/10.1021/mz300585q
Battigelli, A., Almeida, B., & Shukla, A. (2022). Recent advances in bioorthogonal click chemistry for biomedical applications. Bioconjugate Chemistry, 33(2), 263-271. https://doi.org/10.1021/acs.bioconjchem.1c00564
Battigelli, A., Almeida, B., & Shukla, A. (2022). Recent advances in bioorthogonal click chemistry for biomedical applications. Bioconjugate Chemistry, 33(2), 263-271. https://doi.org/10.1021/acs.bioconjchem.1c00564
Chen, Y., Wu, K., Tang, J., Loredo, A., Clements, J., Pei, J., … & Xiao, H. (2019). Addition of isocyanide-containing amino acids to the genetic code for protein labeling and activation. Acs Chemical Biology, 14(12), 2793-2799. https://doi.org/10.1021/acschembio.9b00678
Devaraj, N. (2018). The future of bioorthogonal chemistry. Acs Central Science, 4(8), 952-959. https://doi.org/10.1021/acscentsci.8b00251
Holloway, J., Wetzel, K., Martens, S., Prez, F., & Meier, M. (2019). Direct comparison of solution and solid phase synthesis of sequence-defined macromolecules. Polymer Chemistry, 10(28), 3859-3867. https://doi.org/10.1039/c9py00558g
Janarthanan, G. and Noh, I. (2018). Click chemistry-based injectable hydrogels and bioprinting inks for tissue engineering applications. Tissue Engineering and Regenerative Medicine, 15(5), 531-546. https://doi.org/10.1007/s13770-018-0152-8
Kumar, A. (2024). Designer nanoreactors for bioorthogonal catalysis. Accounts of Chemical Research, 57(3), 413-427. https://doi.org/10.1021/acs.accounts.3c00735
Li, H., Aneja, R., & Chaiken, I. (2013). Click chemistry in peptide-based drug design. Molecules, 18(8), 9797-9817. https://doi.org/10.3390/molecules18089797
Li, J. and Chen, P. (2016). Development and application of bond cleavage reactions in bioorthogonal chemistry. Nature Chemical Biology, 12(3), 129-137. https://doi.org/10.1038/nchembio.2024
Li, J., Kong, H., Zhu, C., & Zhang, Y. (2020). Photo-controllable bioorthogonal chemistry for spatiotemporal control of bio-targets in living systems. Chemical Science, 11(13), 3390-3396. https://doi.org/10.1039/c9sc06540g
Liu, X., Gaihre, B., Li, L., Rezaei, A., Tilton, M., Elder, B., … & Lu, L. (2023). Bioorthogonal “click chemistry” bone cement with bioinspired natural mimicking microstructures for bone repair. Acs Biomaterials Science & Engineering, 9(3), 1585-1597. https://doi.org/10.1021/acsbiomaterials.2c01482
Mueller, E., Poulin, I., Bodnaryk, W., & Hoare, T. (2022). Click chemistry hydrogels for extrusion bioprinting: progress, challenges, and opportunities. Biomacromolecules, 23(3), 619-640. https://doi.org/10.1021/acs.biomac.1c01105
Nebhani, L. and Barner‐Kowollik, C. (2009). Orthogonal transformations on solid substrates: efficient avenues to surface modification. Advanced Materials, 21(34), 3442-3468. https://doi.org/10.1002/adma.200900238
Patel, P., Rana, N., Patel, M., Kozuch, S., & Sabatino, D. (2015). Nucleic acid bioconjugates in cancer detection and therapy. Chemmedchem, 11(3), 252-269. https://doi.org/10.1002/cmdc.201500502
Pałasz, A. (2016). Recent advances in inverse-electron-demand hetero-diels–alder reactions of 1-oxa-1,3-butadienes. Topics in Current Chemistry, 374(3). https://doi.org/10.1007/s41061-016-0026-2
Pookpanratana, S., Savchenko, I., Natoli, S., Cummings, S., Richter, L., Robertson, J., … & Hacker, C. (2014). Attachment of a diruthenium compound to au and sio2/si surfaces by “click” chemistry. Langmuir, 30(34), 10280-10289. https://doi.org/10.1021/la501670c
Row, R. and Prescher, J. (2018). Constructing new bioorthogonal reagents and reactions. Accounts of Chemical Research, 51(5), 1073-1081. https://doi.org/10.1021/acs.accounts.7b00606
Shieh, P. and Bertozzi, C. (2014). Design strategies for bioorthogonal smart probes. Organic & Biomolecular Chemistry, 12(46), 9307-9320. https://doi.org/10.1039/c4ob01632g
Sumerlin, B. and Vogt, A. (2009). Macromolecular engineering through click chemistry and other efficient transformations. Macromolecules, 43(1), 1-13. https://doi.org/10.1021/ma901447e
Taiariol, L., Chaix, C., Farre, C., & Moreau, E. (2021). Click and bioorthogonal chemistry: the future of active targeting of nanoparticles for nanomedicines?. Chemical Reviews, 122(1), 340-384. https://doi.org/10.1021/acs.chemrev.1c00484
Tonga, G., Jeong, Y., Duncan, B., Mizuhara, T., Mout, R., Das, R., … & Rotello, V. (2015). Supramolecular regulation of bioorthogonal catalysis in cells using nanoparticle-embedded transition metal catalysts. Nature Chemistry, 7(7), 597-603. https://doi.org/10.1038/nchem.2284
Tu, J., Xu, M., & Franzini, R. (2019). Dissociative bioorthogonal reactions. Chembiochem, 20(13), 1615-1627. https://doi.org/10.1002/cbic.201800810
Völker, T., Dempwolff, F., Graumann, P., & Meggers, E. (2014). Progress towards bioorthogonal catalysis with organometallic compounds. Angewandte Chemie, 53(39), 10536-10540. https://doi.org/10.1002/anie.201404547
Xie, X., Li, B., Wang, J., Zhan, C., Huang, Y., Zeng, F., … & Wu, S. (2019). Tetrazine-mediated bioorthogonal system for prodrug activation, photothermal therapy, and optoacoustic imaging. Acs Applied Materials & Interfaces, 11(45), 41875-41888. https://doi.org/10.1021/acsami.9b13374
Yao, T., Xu, X., & Huang, R. (2021). Recent advances about the applications of click reaction in chemical proteomics. Molecules, 26(17), 5368. https://doi.org/10.3390/molecules26175368
Zhang, X., Gao, J., Tang, Y., Yu, J., Liew, S., Qiao, C., … & Wang, Z. (2022). Bioorthogonally activatable cyanine dye with torsion-induced disaggregation for in vivo tumor imaging. Nature Communications, 13(1). https://doi.org/10.1038/s41467-022-31136-3
Zhong, X., Yan, J., Ding, X., Chen, S., Xu, Y., & Yang, M. (2023). Recent advances in bioorthogonal click chemistry for enhanced pet and spect radiochemistry. Bioconjugate Chemistry, 34(3), 457-476. https://doi.org/10.1021/acs.bioconjchem.2c00583