High-Resolution 3D Printing Across Disciplines
This review examines high-resolution 3D printing, focusing on technological developments, material diversity, and transformational applications in domains like as health and electronics, as well as anticipating future innovations and problems in precision manufacturing.
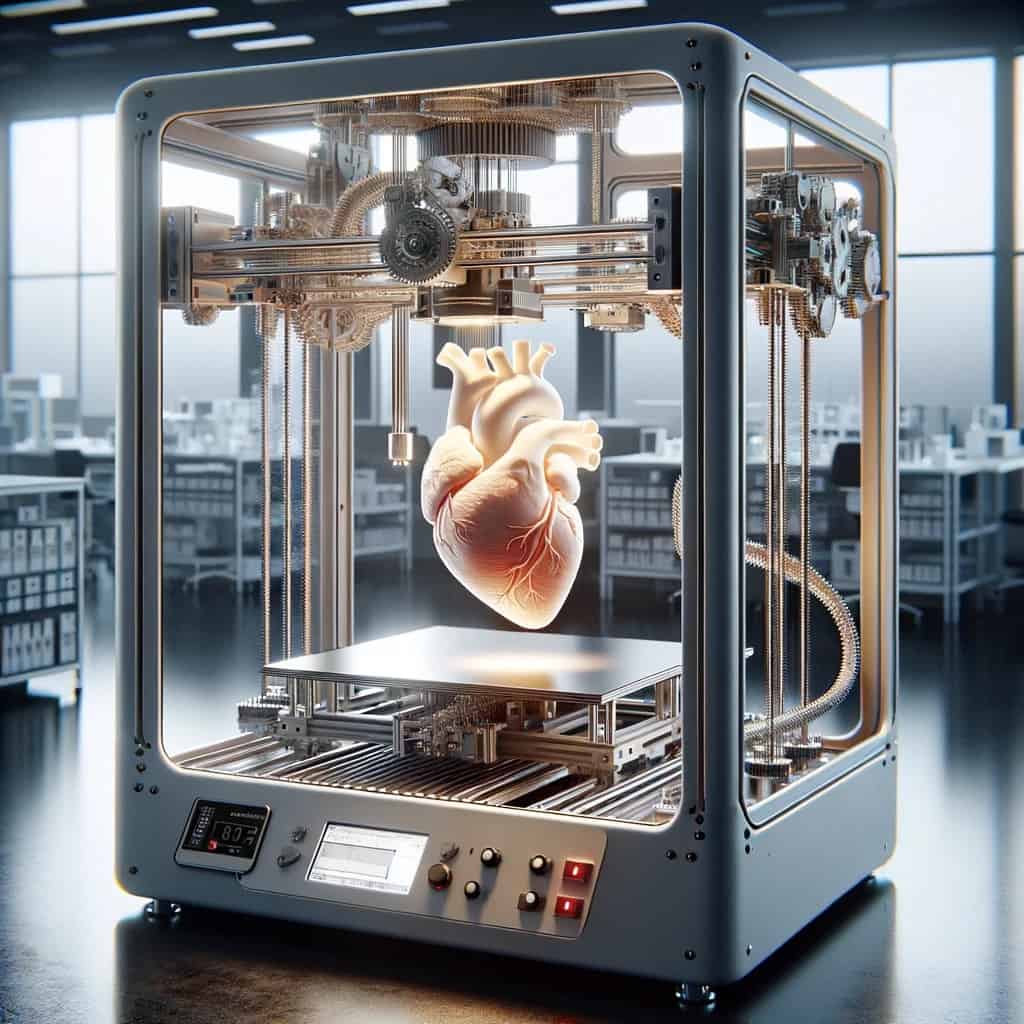
Introduction to High-Resolution 3D Printing Technologies
High-resolution 3D printing technology has evolved greatly in a variety of fields, including health, fashion, and industry. Wang and Yang (2021) examine the use of 3D printing in implantable medical devices, emphasizing the technology’s ability to produce complex structures for medicinal applications. This emphasizes the precision and customisation potential of 3D printing in the healthcare industry. Similarly, Wang et al. (2020) discuss the growing usage of 3D printing in biomedicine due to its potential for quick prototyping and cost-effectiveness, emphasizing its importance in developing personalised solutions.
Kim et al. (2017) used 3D printing technology to assess the shear strength of beams, demonstrating the integration of concrete materials in additive manufacturing processes. This exhibits 3D printing’s versatility in producing structural components with precise mechanical qualities. Furthermore, Wu et al. (2022) investigate the use of 3D printing in smart clothing, demonstrating how the technology may combine garment design with electronic functionality, suggesting its potential to create unique wearable items.
The educational sector also benefits from improvements in 3D printing. Dean et al. (2016) provide hand-held 3D printing pens for teaching VSEPR theory, demonstrating how this technology can improve learning experiences by generating physical models. Furthermore, Lemu and Mikkelsen (2021) examine the use of 3D printing in engineering education, emphasizing its function in quick prototyping and translating digital designs into actual items to improve comprehension.
Furthermore, 3D printing has an impact on several specialist professions, like medicine and ophthalmology. Dong et al. (2022) demonstrate the effectiveness of 3D printing-assisted procedures in difficult renal calculi cases, showing the technology’s potential to improve surgical outcomes. Canabrava et al. (2015) address the use of 3D printing to manufacture intraocular devices, demonstrating how this revolutionary technology is transforming ophthalmic therapies by providing cost-effective and customizable options.
The advancement of high-resolution 3D printing technology has transformed numerous industries by allowing for precise manufacturing, customisation, and rapid prototyping. From healthcare to education and beyond, the importance of 3D printing in generating complicated structures and useful items is clear, paving the way for new applications and breakthroughs in a variety of industries.
Materials and Methods in High-Resolution 3D Printing
High-resolution 3D printing technologies have broadened the spectrum of materials suitable for additive manufacturing procedures. Several studies have looked into novel materials and methods for improving the precision and capabilities of high-resolution 3D printing processes like stereolithography (SLA), digital light processing (DLP), and two-photon polymerization (2PP).
Li et al. (2019) describe the creation of a self-healing polyurethane elastomer based on a photopolymer resin by digital light processing (DLP) 3D printing. This research focuses on the development of materials with unique features appropriate for high-resolution printing applications. Han et al. (2018) investigate soft robotic manipulation with a 3D printed electroactive hydrogel, highlighting the adaptability of materials such as hydrogels in high-resolution 3D printing for sophisticated applications.
Furthermore, Gastaldi et al. (2023) investigate the 4D printing of light-activated shape memory polymers, demonstrating the use of organic dyes in light-based 3D printing techniques such as DLP. Zhou et al. (2022) investigate the use of DLP printing to fabricate ceramic metamaterials for electromagnetic wave absorption, demonstrating the level of precision possible with this technique.
Deng et al. (2019) examine rapid open-air digital light 3D printing of thermoplastic polymers, focusing on the problems and potential associated with printing such materials at high resolution. Nguyen (2024) investigates the effect of graphene oxide and photoinitiator concentrations on the curing behavior of PEG-based polymers for DLP 3D printing, demonstrating the importance of material composition in achieving desired printing results.
Finally, the vast selection of materials suitable with high-resolution 3D printing, ranging from self-healing polymers to ceramic metamaterials, demonstrates additive manufacturing’s ongoing innovation and growth. These findings help to increase the possibilities of high-precision 3D printing technology in a variety of sectors, including robotics and electromagnetic applications.
Applications and Future Directions
High-resolution 3D printing has had a substantial impact on a variety of areas due to its precision and versatility. Yuk et al. (2020) explore the various applications of conducting polymers in energy storage, flexible electronics, and bioelectronics, demonstrating the versatility of high-resolution 3D printing materials. Mao et al. (2017) discuss the typical uses of high-resolution 3D printing, including metamaterials, energy storage, flexible electronics, and microscale tissue engineering scaffolds.
In the medical industry, 3D printing has enabled the creation of unique implants, medicines, biosensors, and tissue engineering scaffolds (Lee et al., 2023). The technology has also enabled the development of patient-specific models for surgical planning and training, considerably advancing healthcare (Qian & Vannan, 2018). Furthermore, shape-memory polymers developed for 4D printing have found applications in aerospace, smart furniture, and soft robotics (Zhang et al., 2021).
High-resolution 3D printing of ceramics, translucent spinel ceramics, and metallic microstructures has created new potential in optics, microelectronics, and terahertz photonics (Wang et al., 2021; Xu et al., 2018; Chen et al., 2022). Furthermore, the combination of high-resolution laser displacement sensors and 3D printing has enhanced structural health monitoring in composite materials and structural engineering (Chang et al., 2017).
Future advances in high-resolution 3D printing include the development of reprocessable thermosets for sustainable printing (Zhang et al., 2018), the engineering of biosensors and biomedical detection devices (Liang et al., 2023), and the investigation of intelligent adjustment of printhead driving waveform parameters for improved print resolution and accuracy (Lin et al., 2017). Continuous innovation in materials, technology, and applications is likely to accelerate high-resolution 3D printing, opening up new opportunities in a variety of industries.
To summarize, high-resolution 3D printing has already achieved substantial progress in a variety of sectors, and continuous research and development are expected to result in promising advances in materials, technology, and applications, cementing 3D printing’s status as a transformative manufacturing technology.
References:
Canabrava, S., Diniz-Filho, A., Schor, P., Fagundes, D., Lopes, A., & Batista, W. (2015). Production of an intraocular device using 3d printing: an innovative technology for ophthalmology. Arquivos Brasileiros De Oftalmologia, 78(6). https://doi.org/10.5935/0004-2749.20150105
Chang, S., Lin, T., Kuo, S., & Huang, T. (2017). Integration of high-resolution laser displacement sensors and 3d printing for structural health monitoring. Sensors, 18(2), 19. https://doi.org/10.3390/s18010019
Chen, H., Chen, X., Lim, J., Lu, Y., Hu, J., Liang, Y., … & Xiao, H. (2022). High‐precision 3d printing of high‐strength polymer‐derived ceramics: impact of precursor’s molecular structure. Advanced Engineering Materials, 24(11). https://doi.org/10.1002/adem.202200269
Dean, N., Ewan, C., & McIndoe, J. (2016). Applying hand-held 3d printing technology to the teaching of vsepr theory. Journal of Chemical Education, 93(9), 1660-1662. https://doi.org/10.1021/acs.jchemed.6b00186
Deng, S., Wu, J., Dickey, M., Zhao, Q., & Xie, T. (2019). Rapid open‐air digital light 3d printing of thermoplastic polymer. Advanced Materials, 31(39). https://doi.org/10.1002/adma.201903970
Dong, C., Fu, Y., Yi, J., Da-li, H., Zhang, Y., Zhang, Z., … & Zhang, B. (2022). Efficacy and safety of 3d printing-assisted percutaneous nephrolithotomy in complex renal calculi. Scientific Reports, 12(1). https://doi.org/10.1038/s41598-021-03851-2
Gastaldi, M., Spiegel, C., Vazquez-Martel, C., Barolo, C., Roppolo, I., & Blasco, E. (2023). 4d printing of light activated shape memory polymers with organic dyes. Molecular Systems Design & Engineering, 8(3), 323-329. https://doi.org/10.1039/d2me00201a
Han, D., Farino, C., Yang, C., Scott, T., Browe, D., Choi, W., … & Lee, H. (2018). Soft robotic manipulation and locomotion with a 3d printed electroactive hydrogel. Acs Applied Materials & Interfaces, 10(21), 17512-17518. https://doi.org/10.1021/acsami.8b04250
Kim, K., Park, S., Kim, W., Jeong, Y., & Lee, J. (2017). Evaluation of shear strength of rc beams with multiple interfaces formed before initial setting using 3d printing technology. Materials, 10(12), 1349. https://doi.org/10.3390/ma10121349
Lee, S., Lee, D., Rajput, N., Levato, T., Shanti, A., & Kim, T. (2023). 3d-printed microcubes for catalase drug delivery. Acs Omega, 8(30), 26775-26781. https://doi.org/10.1021/acsomega.3c00789
Lemu, H. and Mikkelsen, O. (2021). Experience in use of 3d printing in engineering education at university of stavanger. Nordic Journal of Stem Education, 5(1). https://doi.org/10.5324/njsteme.v5i1.3934
Li, X., Yu, R., He, Y., Zhang, Y., Yang, X., Zhao, X., … & Huang, W. (2019). Self-healing polyurethane elastomers based on a disulfide bond by digital light processing 3d printing. Acs Macro Letters, 8(11), 1511-1516. https://doi.org/10.1021/acsmacrolett.9b00766
Liang, M., Liu, F., Chong, Y., Ye, Z., Zhao, L., Q, Y., … & Hu, B. (2023). Engineering biosensors and biomedical detection devices from 3d-printed technology. Ecs Sensors Plus, 2(3), 030604. https://doi.org/10.1149/2754-2726/ace5bd
Lin, N., Jing, S., Chen, H., & Liu, W. (2017). Intelligent adjustment of printhead driving waveform parameters for 3d electronic printing. Matec Web of Conferences, 100, 03034. https://doi.org/10.1051/matecconf/201710003034
Mao, M., He, J., Li, X., Zhang, B., Qi, L., Liu, Y., … & Li, D. (2017). The emerging frontiers and applications of high-resolution 3d printing. Micromachines, 8(4), 113. https://doi.org/10.3390/mi8040113
Nguyen, M. (2024). Role of go and photoinitiator concentration on curing behavior of peg-based polymer for dlp 3d printing. Acs Omega. https://doi.org/10.1021/acsomega.3c05378
Qian, Z. and Vannan, M. (2018). Personalized treatment planning for heart interventions using tissue-mimicking 3d printing. Journal of 3d Printing in Medicine, 2(2), 43-46. https://doi.org/10.2217/3dp-2017-0024
Wang, H., Liu, L., Ye, P., Huang, Z., Ng, A., Du, Z., … & Gan, C. (2021). 3d printing of transparent spinel ceramics with transmittance approaching the theoretical limit. Advanced Materials, 33(15). https://doi.org/10.1002/adma.202007072
Wang, J., Yang, B., Lin, X., Gao, L., Liu, T., Lu, Y., … & Wang, R. (2020). Research of tpu materials for 3d printing aiming at non-pneumatic tires by fdm method. Polymers, 12(11), 2492. https://doi.org/10.3390/polym12112492
Wang, Z. and Yang, Y. (2021). Application of 3d printing in implantable medical devices. Biomed Research International, 2021, 1-13. https://doi.org/10.1155/2021/6653967
Wu, S., Zeng, T., Liu, Z., Guo, M., Xiong, Z., Zuo, L., … & Zhou, Z. (2022). 3d printing technology for smart clothing: a topic review. Materials, 15(20), 7391. https://doi.org/10.3390/ma15207391
Xu, J., Li, X., Zhong, Y., Qi, J., Wang, Z., Chai, Z., … & Cheng, Y. (2018). Glass‐channel molding assisted 3d printing of metallic microstructures enabled by femtosecond laser internal processing and microfluidic electroless plating. Advanced Materials Technologies, 3(12). https://doi.org/10.1002/admt.201800372
Yuk, H., Lu, B., Shen, L., Qu, K., Xu, J., Luo, J., … & Zhao, X. (2020). 3d printing of conducting polymers. Nature Communications, 11(1). https://doi.org/10.1038/s41467-020-15316-7
Zhang, B., Kowsari, K., Serjouei, A., Dunn, M., & Ge, Q. (2018). Reprocessable thermosets for sustainable three-dimensional printing. Nature Communications, 9(1). https://doi.org/10.1038/s41467-018-04292-8
Zhang, B., Li, H., Cheng, J., Ye, H., Sakhaei, A., Yuan, C., … & Ge, Q. (2021). Mechanically robust and uv‐curable shape‐memory polymers for digital light processing based 4d printing. Advanced Materials, 33(27). https://doi.org/10.1002/adma.202101298
Zhou, R., Wang, Y., Liu, Z., Pang, Y., Chen, J., & Kong, J. (2022). Digital light processing 3d-printed ceramic metamaterials for electromagnetic wave absorption. Nano-Micro Letters, 14(1). https://doi.org/10.1007/s40820-022-00865-x