Chitosan Biomaterials: Versatility and Future Directions
While addressing manufacturing issues and projecting future research directions, this review investigates chitosan-based biomaterials, stressing their varied uses in medicine, environmental science, and agriculture.
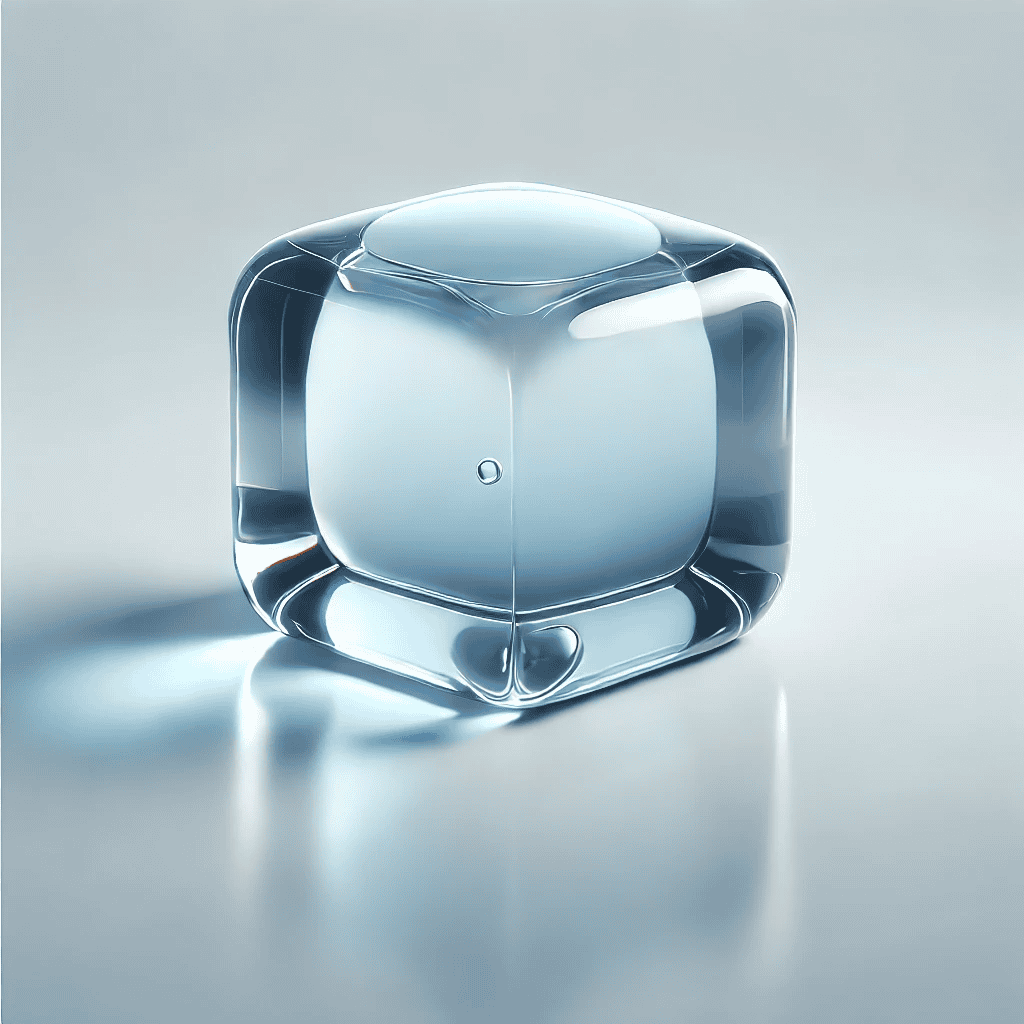
Introduction to Chitosan
Originally generated from chitin, mostly from the exoskeletons of crustaceans such as shrimp, crab, and lobster, chitosan is a naturally occurring biopolymer (Barbusiński et al., 2016). Extracted from clam shells or fungus cultivated in bioreactors, this chitin-derived fiber is also generated from Considered to be antibacterial, chitosan can be chemically altered to improve its qualities for different uses (Shanmugam et al., 2016). In biomaterials research, especially in tissue engineering, this flexible material is advantageous when combined with natural and synthetic polymers as well as other materials ( Kołodziejska et al., 2021).
Chitosan’s special cationic mucoadhesive character and the presence of reactive amino and hydroxyl functional groups make it a desirable biopolymer for drug delivery systems (Andrade et al., 2011; Assa et al., 2016). Moreover, chitosan’s abundance in biomass resources and physicochemical characteristics have attracted research in several disciplines, including fuel cell uses (Ahmed et al., 2023). The degree of deacetylation and acetylation patterns of chitosan determines its applications and characteristics very much (Amamou, 2024; Singh, 2023).
Chitosan has become well-known in biomaterials science because of its biocompatibility and capacity to create hydrogenic and ionic interactions with drug molecules, thereby facilitating drug transport, cancer therapy, and wound healing uses (Bahralouei & Rahman, 2022). Improved solubility for siRNA distribution and enhanced antithrombogenic qualities (Liu et al., 2006; Al-Absi et al., 2022) are two applications of the chemical changes of chitosan that have extended their use. Furthermore created for biomedical uses are chitosan-based scaffolds with improved features, so highlighting the material’s possibilities in tissue engineering (Деминa et al., 2016; Spin‐Neto et al., 2009).
Leveraged for a variety of uses, including purification of effluent and the creation of novel materials, Chitosan’s interactions with metal ions have helped to shape both Furthermore under research are chitosan-apatite composites’ antibacterial qualities and uses in controlled drug delivery systems (Sukhodub et al., 2016). The adaptability of this biomaterial is shown by the investigation of depolymerization of chitosan to change its features for certain uses (Ma et al., 2012).
Ultimately, in biomaterials research, chitosan’s natural supplies, chemical structure, and special characteristics have positioned it as a useful material. From medication delivery systems to tissue engineering, its biocompatibility, antibacterial qualities, and chemical modification ability make it a flexible biopolymer with uses.
Applications of Chitosan-Based Biomaterials
Biomaterials based on chitosan have found extensive use in many different disciplines. Chitosan-based nanoparticles have been applied in agriculture as pesticides, herbicides, and insecticides, therefore enhancing crop quality and productivity. Bandara et al. 2020. Furthermore investigated for sustainable agriculture are chitosan nanoparticle-based delivery systems using nanocomposite films showing antibacterial action against pathogens (Kashyap et al., 2015). In terms of plant protection as well, chitosan has demonstrated potential to improve shelf life and crop quality (Malerba, 2018).
Chitosan-based systems have shown notable antibacterial activity in the biomedical domain, hence they are important for many different medical uses (Atay, 2019). Furthermore suggested for use in tissue engineering, drug transport, and wound healing is chitosan because of its biocompatibility and wound-healing qualities (Atay, 2019). These properties have made chitosan a flexible biomaterial with uses in medicines and wound healing rather interesting.
Moreover, chitosan has been identified as a tool for sustainable development since its uses support sustainability by means of chitosan and its derived components (Maliki et al., 22211). This emphasizes the need of chitosan in supporting environmentally friendly methods and uses.
All things considered, chitosan-based biomaterials have shown promise in sustainability-oriented, biomedical, and agricultural uses. From improving crop security and quality in agriculture to enabling wound healing and drug delivery in the medical industry, chitosan’s adaptability and positive qualities make it a valuable substance across many disciplines.
Challenges and Future Prospects
Although chitosan-based biomaterials have great promise in many different uses, their manufacturing and application present difficulties that have to be resolved if we are to advance. Improving the biocompatibility and biodegradability of chitosan-based biomaterials is therefore quite important to guarantee their safety and efficacy in biomedical uses (Douette et al., 2020). Successful use of biomaterials in host tissues depends on an awareness of their biological reaction in those tissues (Douette et al., 2020). Furthermore, it is still difficult to improve the mechanical qualities of chitosan, such ductility, which calls for careful attention to increase its biomaterial use (Kolhe & Kannan, 2002).
Within the realm of tissue engineering, the creation of chitosan-based scaffolds for regenerative medicine poses issues with cell adhesion and scaffold-cell interactions (Rodríguez-Vázquez et al., 2015). Combining chitosan with other biomaterials such collagen or fibronectin has been proposed to improve cell affinity and scaffold performance (Rodríguez-Vázquez et al., 2015). Furthermore investigated to solve issues with the brittle nature of chitosan films and membrane permeability (Kolhe & Kannan, 2002) is the modification of chitosan using blending and copolymerization with polyethylene glycol.
Research on chitosan biomaterials has future opportunities include investigating fresh approaches for manufacturing chitosan-containing materials for dental and implant engineering uses (Sharifianjazi et al., 2022). Particularly in critical-sized flaws, the creation of chitosan-based scaffolds mixed with additional biomaterials or bioactive compounds shows promise in boosting their regeneration capacity (Signorini, 2023). Furthermore, the use of chitosan-based biomaterials in tissue regeneration initiatives—such as in skin tissue engineering—opens chances to progress regenerative medicine (Chaudhari et al., 2016).
In summary, even if chitosan-based biomaterials have many uses in biomedical, environmental, and agricultural sectors, their continued development depends on addressing issues with biocompatibility, mechanical properties, and scaffold-cell interactions even if they have different applications in these domains. Unlocking the full potential of chitosan-based biomaterials in different uses depends on continuous research projects concentrated on improving their functional qualities and adaptability.
References
Ahmed, S., Tao, Z., Zhang, H., Hassan, M., Ahmed, N., Javid, M., … & Wang, J. (2023). Review on chitosan and two-dimensional mos2-based proton exchange membrane for fuel cell application: advances and perspectives. Energy & Fuels, 37(3), 1699-1730. https://doi.org/10.1021/acs.energyfuels.2c03660
Al-Absi, M., Caprifico, A., & Calabrese, G. (2022). Chitosan and its structural modifications for sirna delivery. Advanced Pharmaceutical Bulletin, 13(2), 275-282. https://doi.org/10.34172/apb.2023.030
Amamou, O. (2024). Revisiting the determination of the degree of deacetylation using potentiometric titration: a new equation for modified chitosan. Molecules, 29(13), 2962. https://doi.org/10.3390/molecules29132962
Andrade, F., Goycoolea, F., Chiappetta, D., Neves, J., & Sosnik, A. (2011). Chitosan-grafted copolymers and chitosan-ligand conjugates as matrices for pulmonary drug delivery. International Journal of Carbohydrate Chemistry, 2011, 1-14. https://doi.org/10.1155/2011/865704
Assa, F., Jafarizadeh‐Malmiri, H., Ajamein, H., Vaghari, H., Anarjan, N., Ahmadi, O., … & Berenjian, A. (2016). Chitosan magnetic nanoparticles for drug delivery systems. Critical Reviews in Biotechnology, 37(4), 492-509. https://doi.org/10.1080/07388551.2016.1185389
Baharlouei, P. and Rahman, M. (2022). Chitin and chitosan: prospective biomedical applications in drug delivery, cancer treatment, and wound healing. Marine Drugs, 20(7), 460. https://doi.org/10.3390/md20070460
Barbusiński, K., Salwiczek, S., & Paszewska, A. (2016). The use of chitosan for removing selected pollutants from water and wastewater – short review. Architecture Civil Engineering Environment, 9(2), 107-115. https://doi.org/10.21307/acee-2016-026
Guibal, E. (2004). Interactions of metal ions with chitosan-based sorbents: a review. Separation and Purification Technology, 38(1), 43-74. https://doi.org/10.1016/j.seppur.2003.10.004
Kołodziejska, M., Jankowska, K., Klak, M., & Wszoła, M. (2021). Chitosan as an underrated polymer in modern tissue engineering. Nanomaterials, 11(11), 3019. https://doi.org/10.3390/nano11113019
Liu, L., Li, F., Fang, Y., & Guo, S. (2006). Regioselective grafting of poly(ethylene glycol) onto chitosan and the properties of the resulting copolymers. Macromolecular Bioscience, 6(10), 855-861. https://doi.org/10.1002/mabi.200600087
Ma, F., Wang, Z., Zhao, H., & Tian, S. (2012). Plasma depolymerization of chitosan in the presence of hydrogen peroxide. International Journal of Molecular Sciences, 13(6), 7788-7797. https://doi.org/10.3390/ijms13067788
Shanmugam, A., Kathiresan, K., & Nayak, L. (2016). Preparation, characterization and antibacterial activity of chitosan and phosphorylated chitosan from cuttlebone of sepia kobiensis (hoyle, 1885). Biotechnology Reports, 9, 25-30. https://doi.org/10.1016/j.btre.2015.10.007
Singh, R. (2023). Unraveling the impact of acetylation patterns in chitosan oligomers on cu2+ ion binding: insights from dft calculations. International Journal of Molecular Sciences, 24(18), 13792. https://doi.org/10.3390/ijms241813792
Spin‐Neto, R., Freitas, R., Pavone, C., Cardoso, M., Filho, S., Marcantonio, R., … & Marcantonio, É. (2009). Histological evaluation of chitosan‐based biomaterials used for the correction of critical size defects in rat’s calvaria. Journal of Biomedical Materials Research Part A, 93A(1), 107-114. https://doi.org/10.1002/jbm.a.32491
Sukhodub, L., Sukhodub, L., & Chorna, I. (2016). Chitosan-apatite composites: synthesis and properties. Biopolymers and Cell, 32(2), 83-97. https://doi.org/10.7124/bc.000910
Vendramin, V., Spinato, G., & Vincenzi, S. (2021). Shellfish chitosan potential in wine clarification.. https://doi.org/10.20944/preprints202102.0555.v1
Демина, Т., Zaytseva-Zotova, D., Акопова, Т., Зеленецкий, А., & Марквичева, Е. (2016). Macroporous hydrogels based on chitosan derivatives: preparation, characterization, and in vitro evaluation. Journal of Applied Polymer Science, 134(13). https://doi.org/10.1002/app.44651
Atay, H. (2019). Antibacterial activity of chitosan-based systems., 457-489. https://doi.org/10.1007/978-981-15-0263-7_15
Bandara, S., Du, H., Carson, L., Bradford, D., & Kommalapati, R. (2020). Agricultural and biomedical applications of chitosan-based nanomaterials. Nanomaterials, 10(10), 1903. https://doi.org/10.3390/nano10101903
Kashyap, P., Xiang, X., & Heiden, P. (2015). Chitosan nanoparticle based delivery systems for sustainable agriculture. International Journal of Biological Macromolecules, 77, 36-51. https://doi.org/10.1016/j.ijbiomac.2015.02.039
Malerba, M. (2018). Recent advances of chitosan applications in plants. Polymers, 10(2), 118. https://doi.org/10.3390/polym10020118
Maliki, S., Sharma, G., Kumar, A., Moral-Zamorano, M., Moradi, O., Baselga, J., … & García‐Peñas, A. (2022). Chitosan as a tool for sustainable development: a mini review. Polymers, 14(7), 1475. https://doi.org/10.3390/polym14071475
Chaudhari, A., Vig, K., Baganizi, D., Sahu, R., Dixit, S., Dennis, V., … & Pillai, S. (2016). Future prospects for scaffolding methods and biomaterials in skin tissue engineering: a review. International Journal of Molecular Sciences, 17(12), 1974. https://doi.org/10.3390/ijms17121974
Douette, P., Chausson, M., Theatre, E., Philippart, C., Gautier, S., Bentin, J., … & Hermitte, L. (2020). Biological safety evaluation of kiomedine® cm-chitosan, an innovative non-animal carboxymethyl-chitosan biomaterial intended for injectable biomedical applications. Journal of Biomaterials, 4(2), 39. https://doi.org/10.11648/j.jb.20200402.12
Kolhe, P. and Kannan, R. (2002). Improvement in ductility of chitosan through blending and copolymerization with peg: ftir investigation of molecular interactions. Biomacromolecules, 4(1), 173-180. https://doi.org/10.1021/bm025689+
Rodríguez-Vázquez, M., Vega-Ruiz, B., Ramos-Zúñiga, R., Saldaña-Koppel, D., & Quiñones-Olvera, L. (2015). Chitosan and its potential use as a scaffold for tissue engineering in regenerative medicine. Biomed Research International, 2015, 1-15. https://doi.org/10.1155/2015/821279
Sharifianjazi, F., Khaksar, S., Esmaeilkhanian, A., Bazli, L., Eskandarinezhad, S., Salahshour, P., … & Vahdat, S. (2022). Advancements in fabrication and application of chitosan composites in implants and dentistry: a review. Biomolecules, 12(2), 155. https://doi.org/10.3390/biom12020155
Signorini, L. (2023). Critical overview on pure chitosan-based scaffolds for bone tissue engineering: clinical insights in dentistry. International Journal of Medical Sciences, 20(12), 1527-1534. https://doi.org/10.7150/ijms.87978