Advances in Sustainable Materials: Synthesis, Applications, and Challenges
This review investigates sustainable materials, with an emphasis on environmentally friendly synthesis, industrial uses, and scaling issues. We highlight technological breakthroughs and suggest future prospects for research and development in this area.
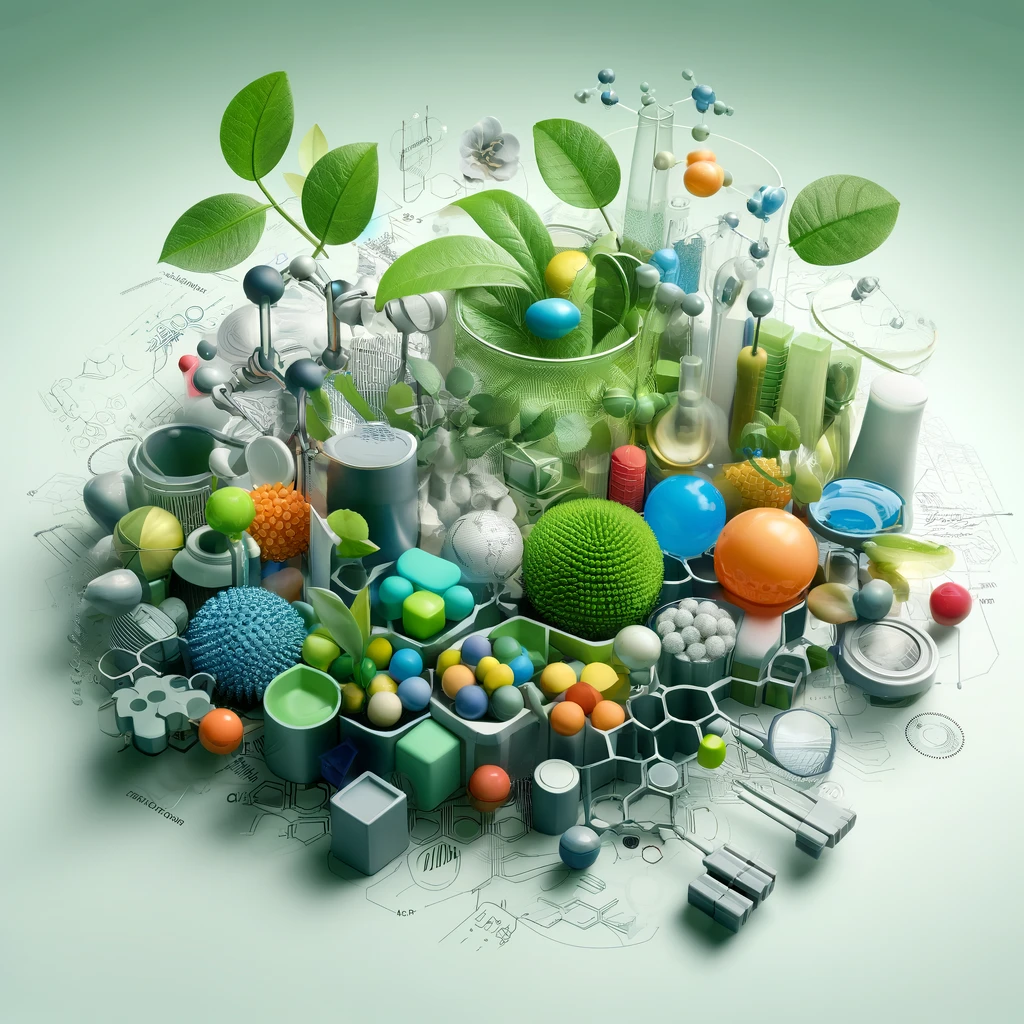
Introduction to Sustainable Materials
Sustainable materials attempt to reduce their environmental impact throughout their life cycle (Baharetha et al., 2012). These materials are important in current science and technology because they have the potential to reduce environmental impact while also giving major economic, social, and environmental benefits (Florez et al., 2013). The concept of sustainability in materials is living comfortably within nature’s means (Milne et al., 2006). Sustainable materials are critical for sustainable development because they help to preserve natural capital while also reducing harm to humanity through effective resource conservation and waste management.
In academia, there have been attempts to define sustainability completely. Sustainable consumption aims to provide basic necessities, improve quality of life, and reduce resource use and waste generation for future generations (Žabkar et al., 2018). Sustainable materials are also essential to sustainable manufacturing techniques, which emphasize effective material utilization as a critical component (Abdul-Rashid & Evans, 2008). Furthermore, the circular economy concept encourages sustainable development by reducing linear material and energy flows via material cycles and renewable energy uses (Tan & Lamers, 2021).
The subject of sustainability reporting and materiality has gained traction in recent years. Materiality in sustainability reporting is described as information that has the potential to significantly influence decisions about sustainable development and value creation, going beyond financial considerations to include broader consequences (Abhayawansa & Adams, 2021). Bridging the gap between financial and social-environmental materiality ideas is critical for effective sustainability reporting (Abhayawansa, 2022). Furthermore, the transition to European sustainability reporting involves a rethinking of the goals and objectives of reporting on sustainability (Baumüller & Sopp, 2021).
Sustainable materials are essential for accomplishing environmental, social, and economic sustainability objectives. Their involvement in a variety of industries, from manufacturing to reporting, emphasizes their importance in encouraging sustainable practices and reducing environmental consequences.
Development and Synthesis of Sustainable Materials
Researchers have investigated a variety of green chemistry-based strategies for synthesizing environmentally friendly polymers and composite materials. Green synthesis of materials, such as silver nanoparticles, has grown in popularity due to its low cost and environmental friendliness, as it eliminates the need for harmful reagents and solvents Mousavi et al. (2018). Furthermore, the insertion of carbon nanotubes into polymer composites using green processing techniques has permitted the production of highly electroconductive materials (Kawamoto & He, 2016). Molecular imprinting techniques have also been used to produce sophisticated materials on a large scale, with an emphasis on practical applications (Arabi et al., 2021).
Green chemistry advances have transformed the production of functionalized nanomaterials, with an emphasis on using environmentally friendly approaches for a wide range of applications (Najeeb et al., 2021). The development of green nanocomposites based on eco-friendly polymers and nanofillers has resulted in the fabrication of green polymeric nanocomposites for energy storage applications. Furthermore, the use of green chemistry concepts, such as aqueous Suzuki-Miyaura cross-coupling reactions, has allowed for the synthesis of biaryls with recyclable precatalysts (Ang, 2020).
Successful applications of sustainable materials include the development of eco-friendly biodegradable composites, such as those reinforced with bagasse fibers, as alternatives to petroleum-based composites (Mehanny et al., 2019). Furthermore, the use of aqueous microwave chemistry has accelerated the production of medicines and fine compounds using sustainable and environmentally friendly protocols (Polshettiwar & Varma, 2008). The stabilization of nanomaterials utilizing naturally available bio-renewable resources has been a step toward greener paths, with an emphasis on sustainability in synthesis and environmental applications.
The synthesis of sustainable materials using eco-friendly methods and green chemistry methodologies offers a possible route to generating environmentally conscious polymers and composites. These innovations not only help to reduce environmental effect, but they also pave the way for new and sustainable material solutions across a wide range of industries.
Applications and Future Perspectives
Key uses of sustainable materials in industries such as automotive, construction, and electronics have played critical roles in advancing environmental sustainability and innovation. In the automotive industry, the use of sustainable materials, such as bio-based composites and recycled plastics, has enabled the production of lightweight and fuel-efficient vehicles, lowering carbon emissions and improving overall sustainability. Niinimäki and Hassi (2011). Similarly, in the construction industry, sustainable materials such as recycled aggregates and eco-friendly concrete have helped to develop energy-efficient structures with low environmental impact (Song et al., 2019). In the electronics industry, the use of sustainable materials such as biodegradable polymers and recyclable components has made it easier to produce eco-friendly electronic gadgets that correspond with global sustainability goals (Vasara et al., 2021).
Despite advancements in utilizing sustainable materials, increasing manufacturing presents considerable obstacles. Cost-effectiveness, scalability of production methods, and maintaining uniform quality standards continue to be significant barriers to large-scale adoption (Liu et al., 2021). To address these problems, viable solutions include streamlining manufacturing processes, investing in R&D for innovative production techniques, and encouraging cross-industry cooperation to streamline supply chains and increase efficiency (Iroegbu & Ray, 2022).
Looking ahead, rising trends and technology in sustainable materials have the potential to change a variety of industries. Advances in multi-material additive manufacturing are projected to encourage the creation of novel materials and structures customized to individual needs, resulting in improved performance and sustainability (Singh et al., 2019). Furthermore, exploring new prospects in functional materials, such as metal phosphonates, shows promise for developing materials with novel functions for a wide range of applications (Zhu et al., 2020). Furthermore, the emphasis on circular economy concepts, bio-derived plastics, and end-of-life strategies demonstrates a move toward sustainable practices and materials management, opening the way for a more environmentally sensitive approach to material use and disposal (Verstraeten et al., 2021).
In conclusion, the use of sustainable materials in the automotive, building, and electronics industries is having a favorable environmental impact. Overcoming production issues through new solutions and embracing future trends in sustainable materials will be critical in creating a more sustainable and environmentally friendly industrial landscape.
Reference
Abdul-Rashid, S. and Evans, S. (2008). A comparison of four sustainable manufacturing strategies. International Journal of Sustainable Engineering, 1(3), 214-229. https://doi.org/10.1080/19397030802513836
Abhayawansa, S. (2022). Swimming against the tide: back to single materiality for sustainability reporting. Sustainability Accounting Management and Policy Journal, 13(6), 1361-1385. https://doi.org/10.1108/sampj-07-2022-0378
Abhayawansa, S. and Adams, C. (2021). Towards a conceptual framework for non-financial reporting inclusive of pandemic and climate risk reporting. Meditari Accountancy Research, 30(3), 710-738. https://doi.org/10.1108/medar-11-2020-1097
Baharetha, S., Al-Hammad, A., & Alshuwaikhat, H. (2012). Towards a unified set of sustainable building materials criteria.. https://doi.org/10.1061/9780784412688.088
Baumüller, J. and Sopp, K. (2021). Double materiality and the shift from non-financial to european sustainability reporting: review, outlook and implications. Journal of Applied Accounting Research, 23(1), 8-28. https://doi.org/10.1108/jaar-04-2021-0114
Florez, L., Castro, D., & Irizarry, J. (2013). Measuring sustainability perceptions of construction materials. Construction Innovation, 13(2), 217-234. https://doi.org/10.1108/14714171311322174
Jones, P., Hillier, D., & Comfort, D. (2016). Sustainability in the hospitality industry. International Journal of Contemporary Hospitality Management, 28(1), 36-67. https://doi.org/10.1108/ijchm-11-2014-0572
Milne, M., Kearins, K., & Walton, S. (2006). Creating adventures in wonderland: the journey metaphor and environmental sustainability. Organization, 13(6), 801-839. https://doi.org/10.1177/1350508406068506
Tan, E. and Lamers, P. (2021). Circular bioeconomy concepts—a perspective. Frontiers in Sustainability, 2. https://doi.org/10.3389/frsus.2021.701509
Žabkar, V., Koklič, M., McDonald, S., & Abosag, I. (2018). Guest editorial. European Journal of Marketing, 52(3/4), 470-475. https://doi.org/10.1108/ejm-04-2018-891
Ang, W. (2020). Integrating green chemistry into teaching laboratories: aqueous suzuki–miyaura cross-coupling reaction using a recyclable fluorous precatalyst. Journal of Chemical Education, 98(1), 203-207. https://doi.org/10.1021/acs.jchemed.0c00072
Arabi, M., Ostovan, A., Li, J., Wang, X., Zhang, Z., Choo, J., … & Chen, L. (2021). Molecular imprinting: green perspectives and strategies. Advanced Materials, 33(30). https://doi.org/10.1002/adma.202100543
Kausar, A. (2021). Green nanocomposites for energy storage. Journal of Composites Science, 5(8), 202. https://doi.org/10.3390/jcs5080202
Kawamoto, M. and He, P. (2016). Green processing of carbon nanomaterials. Advanced Materials, 29(25). https://doi.org/10.1002/adma.201602423
Mehanny, S., Lamis, D., El, H., Emad, E., Mahmoud, F., & Ibrahim, H. (2019). Effect of glue and temperatures on mechanical properties of starch-based biodegradable composites reinforced with bagasse fibers. Int J Biotechnol Biomater Eng, 1(1). https://doi.org/10.35840/2633-8920/8802
Mousavi, S., Hashemi, S., Ghasemi, Y., Atapour, A., Amani, A., Dashtaki, A., … & Arjmand, O. (2018). Green synthesis of silver nanoparticles toward bio and medical applications: review study. Artificial Cells Nanomedicine and Biotechnology, 46(sup3), 855-872. https://doi.org/10.1080/21691401.2018.1517769
Najeeb, J., Naeem, S., Nazar, M., Naseem, K., & Shehzad, U. (2021). Green chemistry: evolution in architecting schemes for perfecting the synthesis methodology of the functionalized nanomaterials. Chemistryselect, 6(13), 3101-3116. https://doi.org/10.1002/slct.202004560
Polshettiwar, V. and Varma, R. (2008). Aqueous microwave chemistry: a clean and green synthetic tool for rapid drug discovery. Chemical Society Reviews, 37(8), 1546. https://doi.org/10.1039/b716534j
Varma, R. (2014). Journey on greener pathways: from the use of alternate energy inputs and benign reaction media to sustainable applications of nano-catalysts in synthesis and environmental remediation. Green Chemistry, 16(4), 2027. https://doi.org/10.1039/c3gc42640h
Iroegbu, A. and Ray, S. (2022). Lignin and keratin-based materials in transient devices and disposables: recent advances toward materials and environmental sustainability. Acs Omega, 7(13), 10854-10863. https://doi.org/10.1021/acsomega.1c07372
Liu, H., Xu, Z., Guo, Z., Feng, J., Li, H., Qiu, T., … & Titirici, M. (2021). A life cycle assessment of hard carbon anodes for sodium-ion batteries. Philosophical Transactions of the Royal Society a Mathematical Physical and Engineering Sciences, 379(2209). https://doi.org/10.1098/rsta.2020.0340
Niinimäki, K. and Hassi, L. (2011). Emerging design strategies in sustainable production and consumption of textiles and clothing. Journal of Cleaner Production. https://doi.org/10.1016/j.jclepro.2011.04.020
Singh, R., Kumar, R., Farina, I., Colangelo, F., Feo, L., & Fraternali, F. (2019). Multi-material additive manufacturing of sustainable innovative materials and structures. Polymers, 11(1), 62. https://doi.org/10.3390/polym11010062
Song, G., Zheng, Y., & Wu, B. (2019). Emerging construction materials and sustainable infrastructure. Applied Sciences, 9(19), 4127. https://doi.org/10.3390/app9194127
Vasara, A., Hakola, L., Välimäki, M., Vilkman, M., Orelma, H., Immonen, K., … & Smolander, M. (2021). 55‐1: invited paper: beyond flexible towards sustainable electronics. Sid Symposium Digest of Technical Papers, 52(1), 764-767. https://doi.org/10.1002/sdtp.14795
Verstraeten, S., Muyden, A., & Bobbink, F. (2021). Towards a plastic circular economy: bio-derived plastics and their end-of-life strategies. Chimia International Journal for Chemistry, 75(9), 744. https://doi.org/10.2533/chimia.2021.744
Zhu, Y., Yuan, Z., & Alshareef, H. (2020). New opportunities for functional materials from metal phosphonates. Acs Materials Letters, 2(6), 582-594. https://doi.org/10.1021/acsmaterialslett.0c00095