Advances in Fluorescent Probes for Enhanced Disease Diagnosis
The evolution of fluorescent probes for illness diagnosis is investigated in this study together with their mechanisms, uses, and future possibilities to improve detection accuracy and specificity in medical diagnosis.
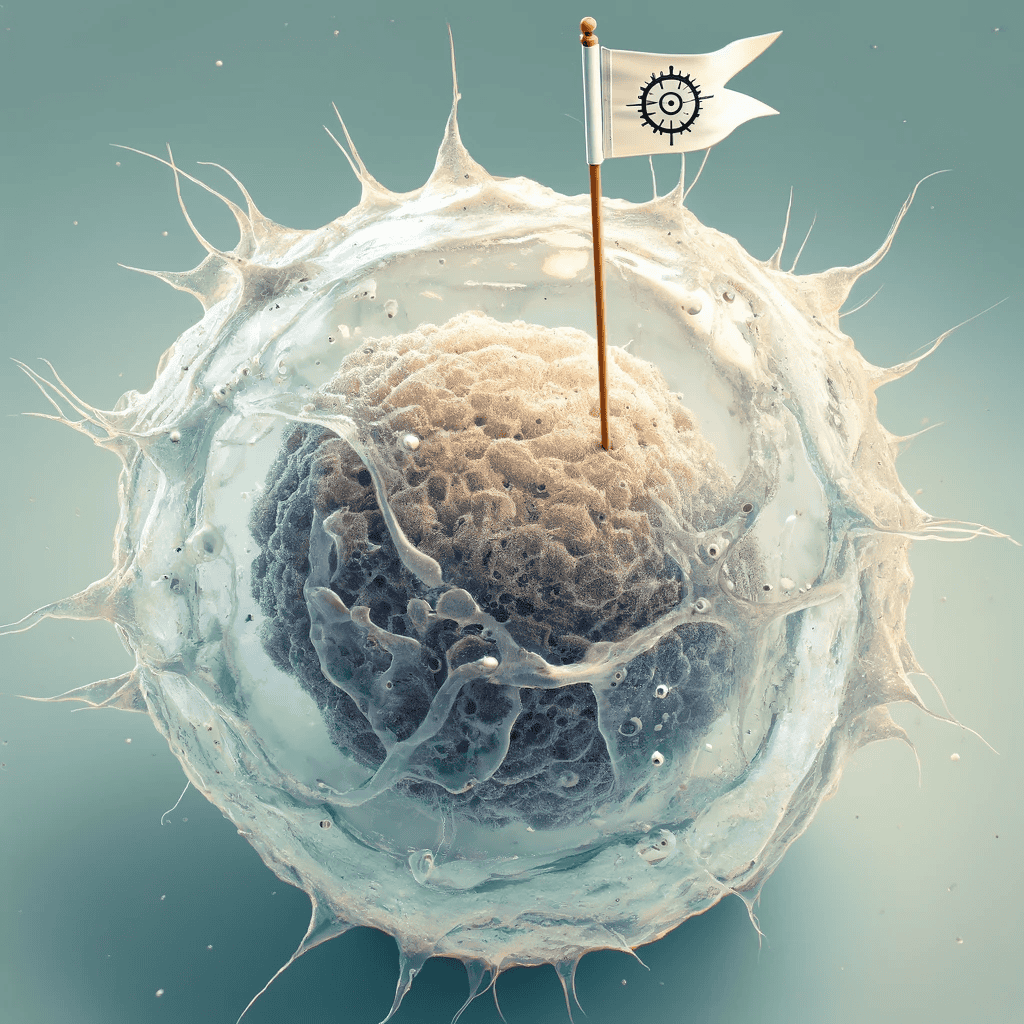
Introduction to Fluorescent Probes
Because of its great sensitivity, selectivity, and capacity to monitor disease-related chemicals at the cellular level and in vivo (Wang, 2024), fluorescent probes have grown indispensable instruments in medical diagnostics. With developments in many fields including DNA fluorescent probes, molecular fluorescence probes, chemical coordination-based probes, and nanoparticle probes, each providing unique operating processes and advantages, these probes have changed dramatically over time (Guo et al., 2020).
Target-specific and enzyme activatable fluorescent probes are absolutely essential in the context of disease detection in allowing the real-time identification of tumor-related chemicals or biological processes within the tumor microenvironment (Hensley et al., 2012). Such probes have showed promise in tracking disease progression related to reactive oxygen species (ROS) and reactive nitrogen species (RNS) and have been quite helpful in identifying chemicals linked with diseases like cancer (Huang et al., 2022).
Particularly in diseases like Alzheimer’s (Yang et al., 2022), the development of near-infrared (NIR) multifunctional fluorescent probes has improved clinical diagnosis capabilities by means of a higher signal-to– background ratio, favorable pharmacokinetics, and noninvasive real-time detection. Fluorescent probes for certain targets, such adenosine triphosphate (ATP), have also shown notable benefits in imaging applications providing high sensitivity, selectivity, real-time imaging, and strong biocompatibility (Gu et al., 2023).
Furthermore, the development of tailored fluorescent probes for enhanced tumor-specific imaging in vivo by means of disease-specific proteins with strong binding affinity linked to fluorescent radiolabels has helped to improve tumor-specific imaging in vivo and so enhance the identification of tumors with great accuracy (Samykutty et al., 2018). These probes highlight the creative methods in probe development since they have been made to overcome obstacles such nonspecific fluorescence and raise detection sensitivity (Zeng et al., 2016).
By providing high sensitivity, selectivity, and real-time imaging features—which have transformed medical diagnostics—fluorescent probes have become indispensable instruments in disease detection and monitoring. From target-specific designs to NIR probes, the constant developments in probe technology emphasize the continued evolution and possibilities of fluorescence probes in improving medical diagnostics.
Mechanisms of Fluorescent Probes in Disease Diagnosis
Essential instruments in illness diagnostics, fluorescent probes interact with disease biomarkers under several processes to generate a fluorescence signal. Different chemical features of these probes—DNA fluorescent probes, molecular fluorescent probes, chemical coordination-based probes, and nanoparticle probes—allow their usefulness (Guo et al., 2020). For in vivo imaging of biomarkers for disease detection, for instance, molecular activatable probes with near-infrared (NIR) fluorescence are very essential, thereby stressing the need of their chemical composition in obtaining precise diagnostic findings (Zeng et al., 2021).
Mechanisms including Förster resonance energy transfer (FRET), photo-induced electron transfer (PET), excited-state intramolecular proton transfer (ESIPT), and enzyme activation (Gao et al., 2017; Chyan & Raines, 2018; Wang et al., 2023; Lu et al., 2022) define the interaction of fluorescent probes with disease biomarkers. These systems let the probes react to analyte contacts, environmental changes, or certain chemical reactions, therefore producing a fluorescence signal in the presence of the target biomarker (Chyan & Raines, 2018). Furthermore, the design of activatable fluorescent probes using both PET and H-dimer synthesis shows improved activation ratios, therefore underlining the need of knowing and applying several mechanisms for best probe performance (Kobayashi & Choyke, 2010).
Furthermore, the development of receptor-targeted fluorescence probes improves our understanding of disease-related receptors, thereby supporting the diagnosis and treatment of many diseases, including malignancies (Zhang et al., 2021), including medication discovery. Using reaction-based fluorescent probes, scientists have precisely identified disease biomarkers, therefore allowing real-time viewing and diagnosis of disorders like malignancies and Alzheimer’s disease (Kim et al., 2023).
Fluorescent probe chemical characteristics define their interactions with illness biomarkers, therefore generating a fluorescent signal for exact disease detection. Design and optimization of fluorescent probes for efficient medical diagnosis depend on an awareness of several mechanisms including FRET, PET, ESIPT, and enzyme activation.
Applications and Future Perspectives
Medical diagnostics have advanced greatly recently with the use of fluorescent probes for the diagnosis of particular disorders such cancer and Alzheimer’s. For chemical biology and disease detection, the creation of enzyme-activated fluorogenic probes, for instance, has made real-time imaging of live cells and in vivo applications possible, therefore offering a useful tool. Furthermore, the construction of near-infrared molecular probes has enabled accurate hepatotoxicity evaluation in vivo, thereby highlighting the possibilities of fluorescence imaging methods for simple, sensitive, noninvasively detecting bioanalytes (Cheng et al., 2019).
Within the field of Alzheimer’s disease, the development of fluorescent probes for amyloid-β aggregates has opened fresh avenues for accurate diagnosis and disease monitoring (Xu et al., 2016). Furthermore, the use of two-photon absorption dyes with low autofluorescence has made in vivo imaging of amyloid-β plaques with minimum background signals possible, hence improving the visualization of important pathogenic indicators in Alzheimer’s disease (Kim et al., 2015).
Regarding cancer diagnosis, the quick imaging of lung cancer employing a red fluorescent probe to identify particular enzyme activity has emphasized the possible of fluorescent probes in the rapid and exact diagnosis of cancer cells (Kawashima et al., 2022). Moreover, the use of fluorescence-guided cancer diagnosis and surgery using zero cross-talk ratiometric near-infrared probes has showed potential in providing particular and sensitive detection of enzyme activity for early cancer diagnosis (Ouyang et al., 2018).
Overcoming obstacles and pushing new boundaries will be part of future developments in probe technology for more general diagnosis purposes. Reaching more selectivity and sensitivity by means of reaction-based fluorescence probes has become a complementary sensing technique to solve the constraints of conventional molecular-recognition-based probes (Singha et al., 2019). Furthermore underlining the continuous attempts to improve diagnosis capabilities using fluorescent probes are the development of multifunctional molecular imaging tools for liver-related diseases and the continuous search of new near-infrared fluorescent probes for Alzheimer’s disease diagnosis (Yang et al., 2022; Xu et al., 2023).
Ultimately, more exact and sensitive diagnostic tools have been made possible by recent advancements in fluorescence probe use for illness diagnosis including cancer and Alzheimer’s. Future developments in probe technology seek to solve problems, including enhancing selectivity and sensitivity, thereby widening the range of diagnostic uses and increasing disease detection capacity.
References:
Gu, Q., Li, T., Liu, T., Yu, G., Mao, G., Xu, F., … & Li, C. (2023). Recent advances in design strategies and imaging applications of fluorescent probes for atp. Chemosensors, 11(7), 417. https://doi.org/10.3390/chemosensors11070417
Guo, J., Yu, H., & Cui, T. (2020). Applications of fluorescent materials in the detection of alkaline phosphatase activity. Journal of Biomedical Materials Research Part B Applied Biomaterials, 109(2), 214-226. https://doi.org/10.1002/jbm.b.34693
Hensley, H., Roder, N., O’Brien, S., Bickel, L., Xiao, F., Litwin, S., … & Connolly, D. (2012). Combined in vivo molecular and anatomic imaging for detection of ovarian carcinoma-associated protease activity and integrin expression in mice. Neoplasia, 14(6), 451-IN2. https://doi.org/10.1596/neo.12480
Huang, T., Yan, S., Yu, Y., Xue, Y., Yu, Y., & Han, C. (2022). Dual-responsive ratiometric fluorescent probe for hypochlorite and peroxynitrite detection and imaging in vitro and in vivo. Analytical Chemistry, 94(2), 1415-1424. https://doi.org/10.1021/acs.analchem.1c04729
Samykutty, A., Thomas, A., McNally, M., Chiba, A., & McNally, L. (2018). Osteopontin-targeted probe detects orthotopic breast cancers using optoacoustic imaging. Biotechnic & Histochemistry, 93(8), 608-614. https://doi.org/10.1080/10520295.2018.1514466
Wang, X. (2024). Fluorescent probes for disease diagnosis. Chemical Reviews. https://doi.org/10.1021/acs.chemrev.3c00776
Yang, Y., Zhang, L., Wang, J., Cao, Y., Li, S., & Liu, Y. (2022). Diagnosis of alzheimer’s disease and in situ biological imaging via an activatable near-infrared fluorescence probe. Analytical Chemistry, 94(39), 13498-13506. https://doi.org/10.1021/acs.analchem.2c02627
Zeng, Y., Gao, R., Wu, T., Cho, C., & Tan, K. (2016). Fluorescent probe encapsulated in snap-tag protein cavity to eliminate nonspecific fluorescence and increase detection sensitivity. Bioconjugate Chemistry, 27(8), 1872-1879. https://doi.org/10.1021/acs.bioconjchem.6b00290
Chyan, W. and Raines, R. (2018). Enzyme-activated fluorogenic probes for live-cell and in vivo imaging. Acs Chemical Biology, 13(7), 1810-1823. https://doi.org/10.1021/acschembio.8b00371
Gao, M., Yu, F., Lv, C., Choo, J., & Chen, L. (2017). Fluorescent chemical probes for accurate tumor diagnosis and targeting therapy. Chemical Society Reviews, 46(8), 2237-2271. https://doi.org/10.1039/c6cs00908e
Guo, J., Yu, H., & Cui, T. (2020). Applications of fluorescent materials in the detection of alkaline phosphatase activity. Journal of Biomedical Materials Research Part B Applied Biomaterials, 109(2), 214-226. https://doi.org/10.1002/jbm.b.34693
Kim, Y., Kim, J., An, J., Park, S., & Kim, D. (2023). All-nontoxic fluorescent probe for biothiols and its clinical applications for real-time glioblastoma visualization. Acs Sensors, 8(4), 1723-1732. https://doi.org/10.1021/acssensors.3c00004
Kobayashi, H. and Choyke, P. (2010). Target-cancer-cell-specific activatable fluorescence imaging probes: rational design and in vivo applications. Accounts of Chemical Research, 44(2), 83-90. https://doi.org/10.1021/ar1000633
Lu, M., Li, Z., Han, C., & Li, W. (2022). New insights into the recognition and sensing mechanism of a h2s fluorescent probe: a theoretical perspective. The Journal of Physical Chemistry A, 126(18), 2788-2793. https://doi.org/10.1021/acs.jpca.2c00782
Wang, W., Wu, W., Wang, M., Liu, Y., Jiao, Y., Wang, F., … & Chen, X. (2023). Excited-state intramolecular proton transfer (esipt)-based fluorescent probes for biomarker detection: design, mechanism, and application. Chemical Communications, 59(15), 2056-2071. https://doi.org/10.1039/d2cc06556h
Zeng, Z., Liew, S., Wei, X., & Pu, K. (2021). Hemicyanine‐based near‐infrared activatable probes for imaging and diagnosis of diseases. Angewandte Chemie, 60(51), 26454-26475. https://doi.org/10.1002/anie.202107877
Zhang, Y., Li, S., Zhang, H., & Xu, H. (2021). Design and application of receptor-targeted fluorescent probes based on small molecular fluorescent dyes. Bioconjugate Chemistry, 32(1), 4-24. https://doi.org/10.1021/acs.bioconjchem.0c00606
Cheng, D., Peng, J., Lv, Y., Su, D., Liu, D., Chen, M., … & Zhang, X. (2019). De novo design of chemical stability near-infrared molecular probes for high-fidelity hepatotoxicity evaluation in vivo. Journal of the American Chemical Society, 141(15), 6352-6361. https://doi.org/10.1021/jacs.9b01374
Chyan, W. and Raines, R. (2018). Enzyme-activated fluorogenic probes for live-cell and in vivo imaging. Acs Chemical Biology, 13(7), 1810-1823. https://doi.org/10.1021/acschembio.8b00371
Kawashima, S., Yoshida, D., Yoshioka, T., Ogasawara, A., Fujita, K., Yanagiya, M., … & Nakajima, J. (2022). Rapid imaging of lung cancer using a red fluorescent probe to detect dipeptidyl peptidase 4 and puromycin-sensitive aminopeptidase activities. Scientific Reports, 12(1). https://doi.org/10.1038/s41598-022-12665-9
Kim, D., Moon, H., Baik, S., Singha, S., Jun, Y., Wang, T., … & Ahn, K. (2015). Two-photon absorbing dyes with minimal autofluorescence in tissue imaging: application to in vivo imaging of amyloid-β plaques with a negligible background signal. Journal of the American Chemical Society, 137(21), 6781-6789. https://doi.org/10.1021/jacs.5b03548
Ouyang, J., Li, Y., Jiang, W., He, S., Liu, H., & Li, C. (2018). Fluorescence-guided cancer diagnosis and surgery by a zero cross-talk ratiometric near-infrared γ-glutamyltranspeptidase fluorescent probe. Analytical Chemistry, 91(1), 1056-1063. https://doi.org/10.1021/acs.analchem.8b04416
Singha, S., Jun, Y., Sarkar, S., & Ahn, K. (2019). An endeavor in the reaction-based approach to fluorescent probes for biorelevant analytes: challenges and achievements. Accounts of Chemical Research, 52(9), 2571-2581. https://doi.org/10.1021/acs.accounts.9b00314
Xu, L., Ma, M., Li, J., Gao, D., Ma, P., Zhang, F., … & Song, D. (2023). Leucine aminopeptidase-mediated multifunctional molecular imaging tool for diagnosis, drug evaluation, and surgical guidance of liver-related diseases. Analytical Chemistry, 95(32), 12089-12096. https://doi.org/10.1021/acs.analchem.3c02130
Xu, M., Ren, W., Tang, X., Hu, Y., & Zhang, H. (2016). Advances in development of fluorescent probes for detecting amyloid-β aggregates. Acta Pharmacologica Sinica, 37(6), 719-730. https://doi.org/10.1038/aps.2015.155
Yang, Y., Zhang, L., Wang, J., Cao, Y., Li, S., & Liu, Y. (2022). Diagnosis of alzheimer’s disease and in situ biological imaging via an activatable near-infrared fluorescence probe. Analytical Chemistry, 94(39), 13498-13506. https://doi.org/10.1021/acs.analchem.2c02627