Advances in Catalytic Water Splitting Technologies
This review investigates the mechanics, catalysts, and problems of water splitting for hydrogen production, emphasizing current advances and future research approaches toward sustainable and efficient energy solutions.
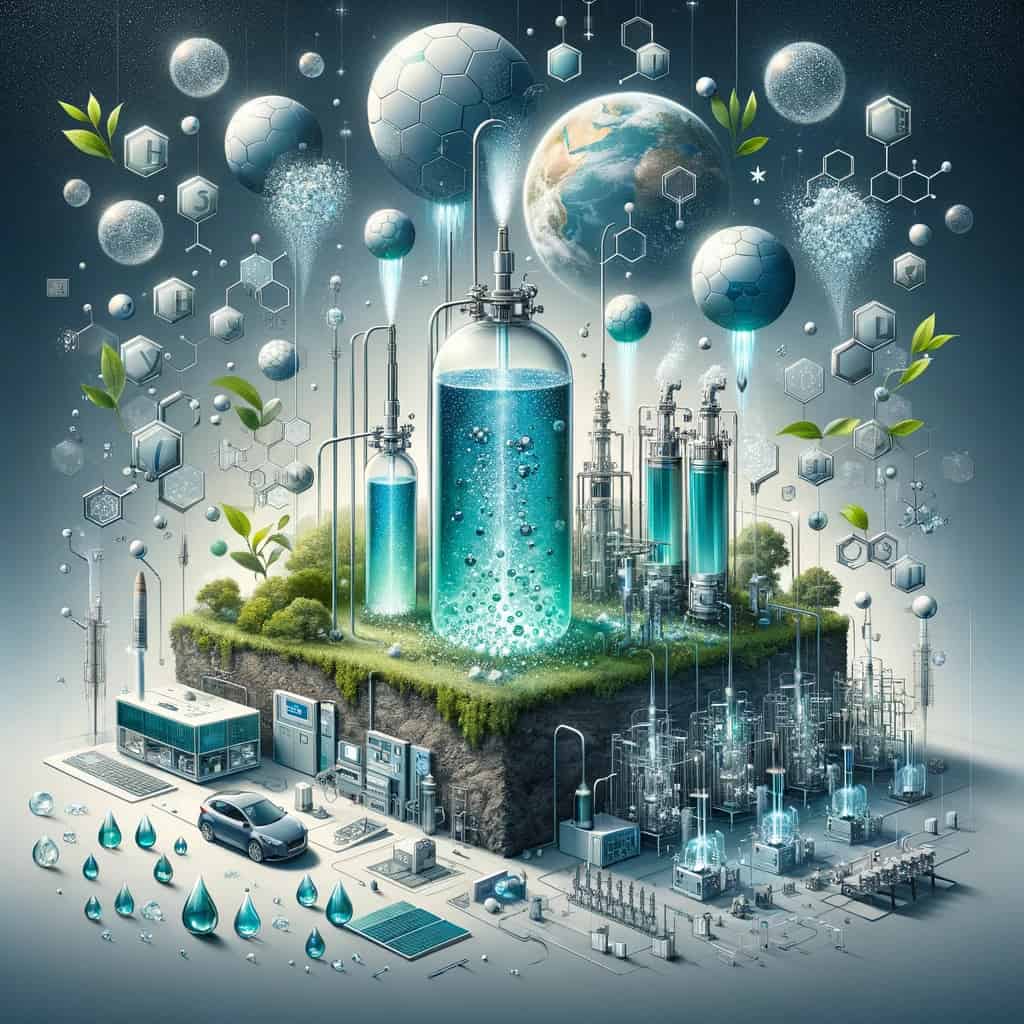
Introduction to Water Splitting
Water splitting, aided by renewable energy sources, is an important step in manufacturing hydrogen, which is seen as an excellent energy source for the future due to its environmental friendliness and sustainability (Ding et al., 2022). The efficiency of water splitting devices is critical for producing green hydrogen from renewable energy sources, since it allows for flexibility in dealing with energy output changes (Passas and Dunnill, 2015). This mechanism consists of two half-reactions: hydrogen evolution at the cathode and oxygen evolution at the anode (Srinivasan et al., 2022).
Catalytic water splitting is significant because of its potential to convert solar energy into hydrogen fuels, providing a clean, carbon-free, and renewable energy source that can help address the environmental and energy concerns connected with fossil fuel consumption (Wu et al., 2015). Electrochemical water splitting, which stores energy as hydrogen and oxygen equivalents, offers a scalable solution for storing renewable energy (Veroneau & Nocera, 2021). Furthermore, water splitting is a viable method for producing hydrogen, particularly when powered by renewable energy sources (Yang et al., 2023).
Photoelectrochemical (PEC) water splitting is a process that uses solar radiation to produce hydrogen from water, providing a mechanism to harness solar energy for long-term hydrogen production (Kumar et al., 2022). Furthermore, metal-free electrocatalyst water splitting is critical for developing clean and sustainable fuel sources (Pradhan & Manna, 2021). The development of improved electrocatalysts, such as Pt-based materials, is critical in increasing the efficiency of the hydrogen evolution reaction in alkaline environments (Ma, 2023).
Solar water splitting, whether via photocatalysis, photovoltaic-electrolysis coupling, or photoelectrochemical approaches, shows promise for the creation of renewable hydrogen (Amika et al., 2022). The use of perovskite-based photoelectrodes has resulted in excellent overall water splitting, indicating the possibility of high solar-to-hydrogen conversion efficiencies (Rhee et al., 2022). Furthermore, developing effective hydrogen evolution catalysts is crucial for promoting electrochemical water splitting as a sustainable energy source (Zhan, 2024).
Water splitting powered by renewable energy sources is an important technique for sustainable hydrogen production, providing a clean and efficient means to address the growing demand for clean energy solutions. Water splitting can help to accelerate the transition to a more sustainable energy future by utilizing a variety of catalytic and electrochemical processes.
Mechanisms and Catalysts
There are two main methods for creating hydrogen: electrolytic and photocatalytic water splitting, each with its own set of properties and significance. Electrolytic water splitting uses electrical energy to drive the disintegration of water into hydrogen and oxygen. This process occurs in an electrolyzer, when water is split into its constituent gases using an electric current. Photocatalytic water splitting, on the other hand, uses light energy to start the process, with semiconductor materials serving as photocatalysts to help split water molecules into hydrogen and oxygen.
Catalysts are essential in both electrolytic and photocatalytic water splitting processes because they increase efficiency and reduce energy requirements. Catalysts are employed in electrolytic water splitting to reduce the overpotential required for hydrogen and oxygen evolution processes, increasing overall process efficiency. Similarly, in photocatalytic water splitting, catalysts are required to promote the separation of photoexcited charge carriers, facilitate redox processes, and increase total hydrogen production efficiency.
Ongoing research is aimed at identifying cost-effective and abundant materials to employ as catalysts in water splitting systems. Metal phosphides, metal-organic frameworks, mixed halide perovskites, and carbon-based structures are among the materials investigated to improve the efficiency of both electrolytic and photocatalytic water splitting. The development of efficient catalysts is critical for developing the science of water splitting and permitting the long-term production of hydrogen as a clean energy carrier.
Electrolytic and photocatalytic water splitting are critical methods for hydrogen production, and catalysts play an important role in increasing efficiency. Ongoing research efforts are aimed at identifying and developing cost-effective and abundant catalyst materials to drive breakthroughs in water splitting and contribute to the transition to a more sustainable energy future.
Challenges and Future Perspectives
Water splitting technologies have numerous problems that limit their general acceptance and commercial viability. These obstacles include scale issues, energy efficiency concerns, and the economic viability of hydrogen fuel production. Landman et al. (2017) state that lowering hydrogen production prices is a significant barrier to the widespread use of sustainable energy technology. Water splitting, which is the energetically uphill reaction of splitting water into hydrogen and oxygen, is a significant technological problem due to the multiproton coupled electron transfer process involved (Panda et al., 2014).
The sustainable production of hydrogen in terms of energy consumption and environmental effect remains a major problem for the evolution of hydrogen-based technologies (Kim et al., 2020). Challenges also arise from the slow kinetics of half-reactions, such as the oxygen evolution reaction (OER) and the hydrogen evolution reaction (HER), which reduce the overall efficiency of water splitting operations. Furthermore, developing durable and efficient catalyst materials for OER remains a critical problem in the advancement of water splitting technologies (Inamdar et al., 2019).
Efforts to overcome these difficulties require interdisciplinary approaches and novel research avenues. The creation of effective bifunctional catalysts capable of initiating both anodic and cathodic processes concurrently is critical for overall water splitting (He et al., 2019). The search for effective noble-metal-free electrodes is critical to developing sustainable hydrogen communities (Jeong et al., 2019). Furthermore, improving high-performance electrocatalysts for the oxygen evolution process (OER) is critical for the development of water splitting technologies (Ma et al., 2023).
Future research should focus on overcoming the slow kinetics of water oxidation, improving gas separation, and increasing the efficiency and durability of catalyst materials. The development of effective catalysts, the investigation of innovative electrode materials, and the tuning of reaction conditions are all critical steps toward developing water splitting technology. By combining skills from multiple fields such as chemistry, materials science, physics, and electrochemistry, researchers can work together to overcome the problems of water splitting and set the groundwork for a sustainable and clean energy future.
References:
Amika, N., Sharma, R., Sharma, A., Chandrani, A., Singh, J., & Kumar, D. (2022). Role of titanate-based perovskites in solar water splitting: an overview. Journal of Physics Conference Series, 2267(1), 012016. https://doi.org/10.1088/1742-6596/2267/1/012016
Chang, K., Tran, D., Wang, J., Kim, N., & Lee, J. (2022). A 3d hierarchical network derived from 2d fe-doped nise nanosheets/carbon nanotubes with enhanced oer performance for overall water splitting. Journal of Materials Chemistry A, 10(6), 3102-3111. https://doi.org/10.1039/d1ta07393a
Ding, Y., Li, N., Yuan, S., & Yu, L. (2022). Surface and interface engineering strategies for mos2 towards electrochemical hydrogen evolution. Chemistry – An Asian Journal, 17(14). https://doi.org/10.1002/asia.202200178
He, M., Feng, C., Liao, T., Hu, S., Wu, H., & Sun, Z. (2019). Low-cost ni2p/ni0.96s heterostructured bifunctional electrocatalyst toward highly efficient overall urea-water electrolysis. Acs Applied Materials & Interfaces, 12(2), 2225-2233. https://doi.org/10.1021/acsami.9b14350
Inamdar, A., Chavan, H., Hou, B., Lee, C., Lee, S., Cha, S., … & Im, H. (2019). A robust nonprecious cufe composite as a highly efficient bifunctional catalyst for overall electrochemical water splitting. Small, 16(2). https://doi.org/10.1002/smll.201905884
Jeong, S., Hu, K., Ohto, T., Nagata, Y., Masuda, H., Fujita, J., … & Ito, Y. (2019). Effect of graphene encapsulation of nimo alloys on oxygen evolution reaction. Acs Catalysis, 10(1), 792-799. https://doi.org/10.1021/acscatal.9b04134
Kim, D., Lee, D., Kim, S., Park, W., & Sim, U. (2020). Photoelectrochemical water splitting reaction system based on metal-organic halide perovskites. Materials, 13(1), 210. https://doi.org/10.3390/ma13010210
Kumar, M., Meena, B., Subramanyam, P., Suryakala, D., & Subrahmanyam, C. (2022). Recent trends in photoelectrochemical water splitting: the role of cocatalysts. NPG Asia Materials, 14(1). https://doi.org/10.1038/s41427-022-00436-x
Landman, A., Dotan, H., Shter, G., Wullenkord, M., Houaijia, A., Maljusch, A., … & Rothschild, A. (2017). Photoelectrochemical water splitting in separate oxygen and hydrogen cells. Nature Materials, 16(6), 646-651. https://doi.org/10.1038/nmat4876
Ma, W. (2023). Advanced pt-based electrocatalysts for the hydrogen evolution reaction in alkaline medium. Nanoscale, 15(28), 11759-11776. https://doi.org/10.1039/d3nr01940c
Ma, X., Xiao, H., Gao, Y., Zhao, M., Zhang, L., Zhang, J., … & Wu, H. (2023). Enhancement of pore confinement caused by the mosaic structure on ru nanoparticles for ph-universal hydrogen evolution reaction. Journal of Materials Chemistry A, 11(7), 3524-3534. https://doi.org/10.1039/d2ta09167d
Panda, C., Debgupta, J., Díaz, D., Singh, K., Gupta, S., & Dhar, B. (2014). Homogeneous photochemical water oxidation by biuret-modified fe-taml: evidence of fev(o) intermediate. Journal of the American Chemical Society, 136(35), 12273-12282. https://doi.org/10.1021/ja503753k
Passas, G. and Dunnill, C. (2015). Water splitting test cell for renewable energy storage as hydrogen gas. Journal of Fundamentals of Renewable Energy and Applications, 05(05). https://doi.org/10.4172/2090-4541.1000188
Pradhan, A. and Manna, R. (2021). Surface-modified covalent organic polymer for metal-free electrocatalytic hydrogen evolution reaction. Acs Applied Polymer Materials, 3(3), 1376-1384. https://doi.org/10.1021/acsapm.0c01238
Rhee, R., Kim, T., Jang, G., Bae, G., Lee, J., Lee, S., … & Park, J. (2022). Unassisted overall water splitting with a solar‐to‐hydrogen efficiency of over 10% by coupled lead halide perovskite photoelectrodes. Carbon Energy, 5(1). https://doi.org/10.1002/cey2.232
Srinivasan, S., Liu, Z., House, S., & Jin, R. (2022). Size-dependent electrocatalytic water oxidation activity for a series of atomically precise nickel-thiolate clusters. Inorganic Chemistry, 62(5), 1875-1884. https://doi.org/10.1021/acs.inorgchem.2c01292
Veroneau, S. and Nocera, D. (2021). Continuous electrochemical water splitting from natural water sources via forward osmosis. Proceedings of the National Academy of Sciences, 118(9). https://doi.org/10.1073/pnas.2024855118
Wu, Y., Chen, M., Han, Y., Luo, H., Su, X., Zhang, M., … & Cao, R. (2015). Fast and simple preparation of iron‐based thin films as highly efficient water‐oxidation catalysts in neutral aqueous solution. Angewandte Chemie, 127(16), 4952-4957. https://doi.org/10.1002/ange.201412389
Yang, W., Qiu, Y., Bao, J., Luo, C., & Zhao, Q. (2023). Thermo-electrochemical redox-mediated decoupling of water splitting for improving h2 production. Industrial & Engineering Chemistry Research, 62(25), 9932-9938. https://doi.org/10.1021/acs.iecr.3c00901
Zhan, J. (2024). Design of high-efficiency hydrogen evolution catalysts in a chiral crystal. Acs Catalysis, 14(2), 1030-1036. https://doi.org/10.1021/acscatal.3c03086