Advances in Biomaterials for Next-Gen Wearable Monitors
The usefulness, user compliance, and general efficacy of wearable health monitoring systems have been improved with the incorporation of cutting-edge biomaterials. This investigation focuses on the characteristics, workings, and effects of advanced biomaterials used in wearable technology.
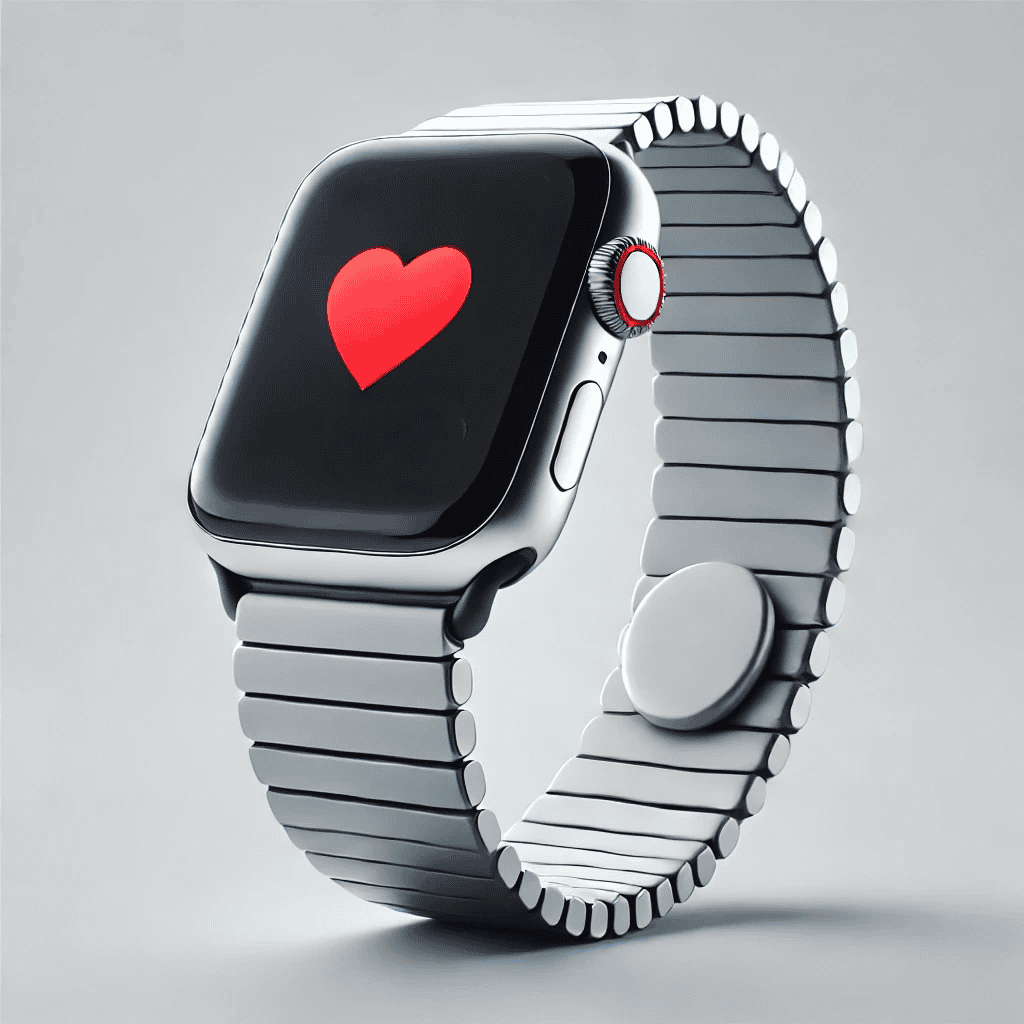
The creation of stretchy piezoelectric sensing systems is among the most noteworthy developments in wearable health monitoring. By using materials that can adapt to the shape of the body, these devices make it possible to monitor physiological signals like heart rate and strain without intrusive procedures. In order to preserve functioning on curved surfaces and wirelessly transfer data via NFC technology, Sun et al., for example, showed a self-powered strain monitoring system that makes use of a kirigami patterning approach (Sun et al., 2019). In addition to improving user comfort, this creative design guarantees continuous monitoring without the need for bulky add-ons.
Furthermore, a significant development in wearable biosensors is the integration of sweat sensing technology. Without requiring human input, which is frequently a drawback of conventional approaches, these devices may measure biomarkers like cortisol, offering real-time information into a person’s stress levels (Samson & Koh, 2020). In order to enable continuous health monitoring and enhance user compliance, Ghaffari et al. stressed the significance of creating dependable biosensing devices that can detect a variety of biochemical signals from perspiration (Ghaffari et al., 2021). These devices are more useful and effective in a variety of settings thanks to the incorporation of flexible substrates and sophisticated signal processing circuits (Qiao et al., 2020).
Wearable technology has embraced the use of biodegradable and biocompatible materials in addition to sweat sensors. In order to increase biocompatibility and lessen the environmental impact of wearable technology, Liu et al. emphasized the potential of employing protein nanowires as sensing elements in humidity sensors (Liu et al., 2020). Since flexible, biodegradable materials can reduce waste and improve user safety, their development is in line with the growing demand for sustainable health monitoring technologies (Wang et al., 2020).
Additionally, it has been demonstrated that the hybrid integration of soft materials with cutting-edge sensing technologies enhances the wearable devices’ mechanical flexibility and usability. The importance of integrating biocompatible materials with miniature wireless systems to improve wearable health monitor performance was covered by Lim et al. (Lim et al., 2019). This integration makes it possible to develop gadgets that are comfortable to use for extended periods of time in addition to being efficient at monitoring health metrics, which increases user compliance.
The development of wearable sensors also heavily depends on the investigation of piezoelectric biomaterials. Recent research has demonstrated the successful use of piezoelectric materials in biosensors and actuators, allowing for the safe integration of these devices with biological systems for a range of uses, such as tissue healing stimulation and physiological force monitoring (Chorsi et al., 2018). The practicality and sustainability of wearable technology are further improved by the capacity to use the mechanical energy produced by body movements to power them (Yan et al., 2021).
In summary, the field of personal health management is changing as a result of continuous advancements in biomaterials for wearable health monitoring devices. Stretchable, biodegradable, and biocompatible materials combined with cutting-edge sensing technologies improve device performance while encouraging environmental sustainability and user compliance. The potential for emerging technologies to enhance user experiences and health outcomes is still encouraging as research advances.
References
Chorsi, M., Curry, E., Chorsi, H., Das, R., Baroody, J., Purohit, P., … & Nguyen, T. (2018). Piezoelectric biomaterials for sensors and actuators. Advanced Materials, 31(1). https://doi.org/10.1002/adma.201802084
Ghaffari, R., Yang, D., Kim, J., Mansour, A., Wright, J., Model, J., … & Ray, T. (2021). State of sweat: emerging wearable systems for real-time, noninvasive sweat sensing and analytics. Acs Sensors, 6(8), 2787-2801. https://doi.org/10.1021/acssensors.1c01133
Lim, H., Kim, H., Qazi, R., Kwon, Y., Jeong, J., & Yeo, W. (2019). Advanced soft materials, sensor integrations, and applications of wearable flexible hybrid electronics in healthcare, energy, and environment. Advanced Materials, 32(15). https://doi.org/10.1002/adma.201901924
Liu, X., Fu, T., Ward, J., Gao, H., Yin, B., Woodard, T., … & Yao, J. (2020). Multifunctional protein nanowire humidity sensors for green wearable electronics. Advanced Electronic Materials, 6(9). https://doi.org/10.1002/aelm.202000721
Qiao, L., Benzigar, M., Subramony, J., Lovell, N., & Liu, G. (2020). Advances in sweat wearables: sample extraction, real-time biosensing, and flexible platforms. Acs Applied Materials & Interfaces, 12(30), 34337-34361. https://doi.org/10.1021/acsami.0c07614
Samson, C. and Koh, A. (2020). Stress monitoring and recent advancements in wearable biosensors. Frontiers in Bioengineering and Biotechnology, 8. https://doi.org/10.3389/fbioe.2020.01037
Sun, R., Carreira, S., Chen, Y., Xiang, C., Xu, L., Zhang, B., … & Rossiter, J. (2019). Stretchable piezoelectric sensing systems for self‐powered and wireless health monitoring. Advanced Materials Technologies, 4(5). https://doi.org/10.1002/admt.201900100
Wang, L., Lou, Z., Wang, K., Zhao, S., Yu, P., Wei, W., … & Shen, G. (2020). Biocompatible and biodegradable functional polysaccharides for flexible humidity sensors. Research, 2020. https://doi.org/10.34133/2020/8716847
Yan, S., Zhang, Z., Shi, X., Xu, Y., Li, Y., Li, Q., … & Turng, L. (2021). Eggshell membrane and expanded polytetrafluoroethylene piezoelectric‐enhanced triboelectric bio‐nanogenerators for energy harvesting. International Journal of Energy Research, 45(7), 11053-11064. https://doi.org/10.1002/er.6589