Advancements and Challenges in Chemical Sterilization
Chemical sterilization is investigated in this review together with mechanisms, main agents, and new trends. It draws attention to difficulties with efficiency, safety, and creativity in both industrial and medical uses.
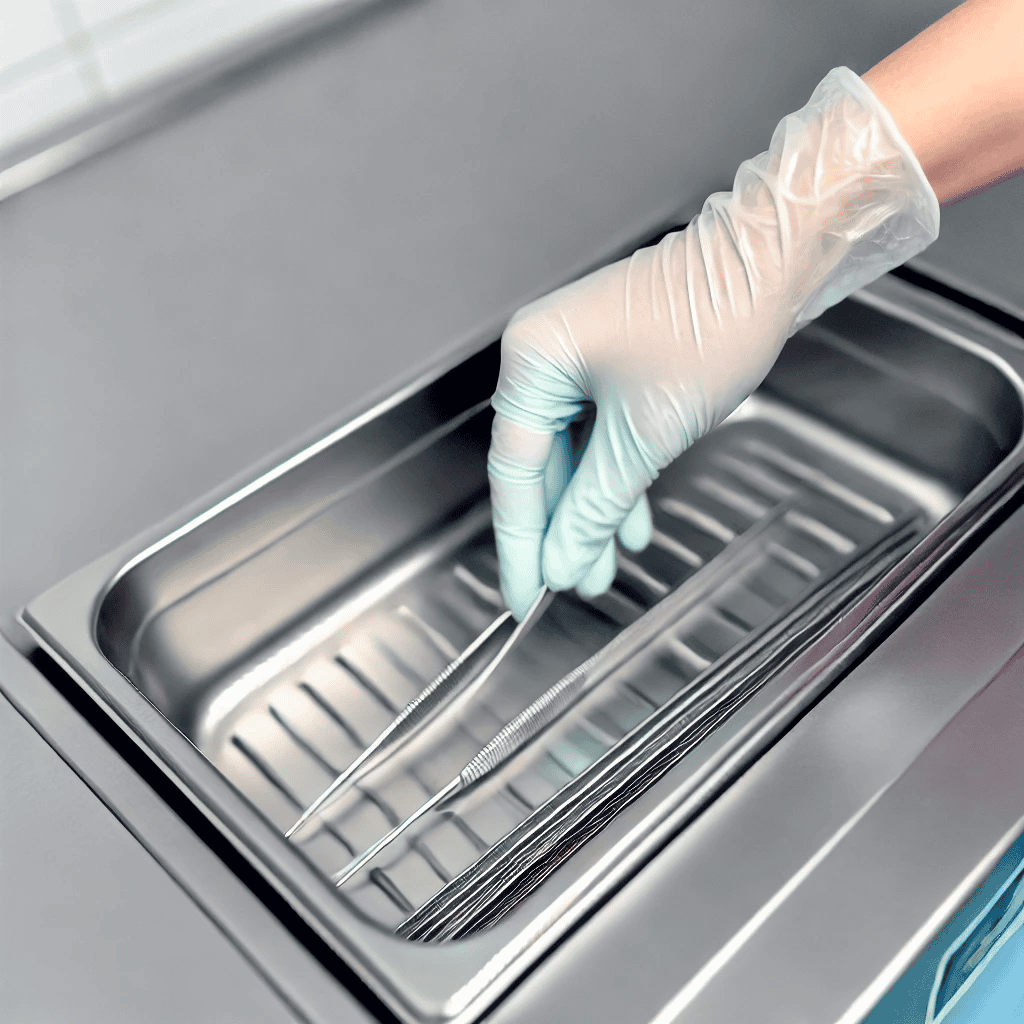
Introduction to Chemical Sterilization
Aimed at eradicating all kinds of microbial life, including bacteria, viruses, fungus, and spores, chemical sterilization is a vital procedure in both industrial and medical uses. This technique offers different benefits over physical techniques as heat or radiation since it uses several chemical agents to reach sterilization.
Chemical sterilization is based on the idea of using chemical agents that either upset microorganisms’ metabolic activities or cellular structure. Common agents working through distinct processes are ethylene oxide (EO), hydrogen peroxide, and chlorine dioxide. EO, for example, alkylates cellular components to inactivate microbial DNA and proteins; hydrogen peroxide creates reactive oxygen species that compromise cell structures (Mansour et al., 2022; Cardoso & Inthurn, 2018). High temperatures could impair several medical devices and biological tissues, hence these chemical techniques are especially helpful for sterilizing heat-sensitive materials (Seif et al., 2022).
Chemical sterilization’s capacity to pass difficult geometries and porous materials guarantees complete sterilization of complex devices such as endoscopes and surgical tools, therefore one of its main benefits (Arif & Delclos, 2011). Chemical sterilization is also appropriate for a greater spectrum of materials, including plastics and delicate equipment unable of withstanding autoclaving (Dai et al., 2016), since it may be carried out at lower temperatures. Moreover, especially when using agents like hydrogen peroxide, which breaks down into water and oxygen after application, so avoiding dangerous residues, chemical sterilizing techniques frequently produce low residual effects (Kurose & Aoyagi, 2016).
Chemical sterilization has special advantages over physical sterilizing techniques including autoclaving or gamma irradiation. For instance, whereas chemical techniques can preserve the integrity of sensitive components (Seif et al., 2022), gamma irradiation may change the physical characteristics of some materials even if it is effective. Furthermore, under some conditions—especially in resource-limited locations where access to sophisticated equipment may be limited—chemical sterilization can be more affordable and simpler to apply (Cardoso & Inthurn, 2018).
All things considered, chemical sterilizing is absolutely essential to guarantee the safety and effectiveness of industrial and medical products. It is a necessary instrument in the fight against infection and contamination since it can efficiently eradicate bacteria while maintaining the integrity of delicate items.
Key Chemical Agents and Mechanisms
A key technique used in many disciplines, especially in industry and healthcare, chemical sterilization guarantees the eradication of all types of microbial life. Each with unique methods of action, efficacy, safety profiles, and environmental implications, among the most often employed chemical agents for sterilization are ethylene oxide (EO), formaldehyde, and glutaraldehyde.
A well-known sterilizing agent, ethylene oxide is very successful against a wide spectrum of microorganisms including bacteria, viruses, and spores. EO operates through alkylation, in which the ethylene oxide molecule interacts with nucleophilic sites on microbial DNA and proteins to cause disturbance of vital biological activities. Cross-links produced by this alkylation mechanism in DNA stop transcription and replication, therefore causing cell death (Iwamoto et al., 2021). Because EO runs efficiently at low temperatures, so preserving the integrity of delicate instruments, it is very helpful for sterilizing heat-sensitive medical devices and materials (Iwamoto et al., 2021).
Regarding effectiveness, EO is regarded as one of the gold standards for low-temperature sterilizing techniques. But its use comes with safety issues since EO is categorized as a human carcinogen and, in case appropriate care is not followed, it might endanger healthcare professionals (Santovito et al., 2013). EO has a major environmental influence as well since it contributes to air pollution and is under strict use and disposal rules (Santovito et al., 2013).
Often employed in the form of formalin, a 37% solution of formaldehyde in water, formaldehyde is another strong chemical sterilizer. Involving the alkylation of proteins and nucleic acids, which results in microbial inactivation, its mechanism of action is like that of EO (Garcia-Haro et al., 2021). Effective against a broad spectrum of pathogens, including bacteria, viruses, and fungi, formaldehyde is especially well-known for its penetrative power, hence fitting for sterilizing complicated medical tools (Garcia-Haro et al., 2021).
Although formaldehyde is useful, it also seriously compromises safety. Classed as a likely human carcinogen, it can irritate the respiratory system and produce allergy responses in exposed people (Santovito et al., 2013). Formaldehyde’s environmental effects also raise questions since it can aggravate indoor air pollution and has been linked to certain health problems in workplaces (Santovito et al., 2013). Furthermore, formaldehyde should be used carefully and under ventilation to reduce exposure hazards (Garcia-Haro et al., 2021).
Another chemical agent used in sterilization is glutaraldehyde, especially in medical environments for the cleaning of tools. Cross-linking proteins under its mechanism of action denaturates microbial cells and finally causes their death (Iwamoto et al., 2021). A useful choice for high-level disinfection (Iwamoto et al., 2021) glutaraldehyde is efficient against a broad spectrum of bacteria, including spores.
Glutaraldehyde is classed as a sensitizer (Santovito et al., 2013) so it also raises safety issues since it might irritate skin and respiratory systems. Though its environmental impact is less clear than that of EO and formaldehyde, glutaraldehyde nevertheless needs cautious management to prevent environmental contamination (Santovito et al., 2013).
When evaluating these chemical agents, one has to take numerous elements into account including environmental effect, safety, and efficacy. Although ethylene oxide has low-temperature functioning and broad-spectrum efficacy, its carcinogenic potential and environmental issues cannot be discounted. Although formaldehyde has comparable effectiveness, especially with continuous exposure it presents major health hazards. Although glutaraldehyde is efficient, it should be handled carefully since it raises safety issues.
Therefore, the particular application, the materials being sterilized, and the balance between efficacy, safety, and environmental issues determine the chemical sterilizing agent to be used. Every agent has advantages and disadvantages; so, knowledge of these elements is crucial for wise judgments in sterilizing procedures.
Challenges and Future Directions
Chemical sterilization’s field of use suffers various difficulties that affect its safety, regulatory compliance, and effectiveness. Important problems are regulatory obstacles, material compatibility, and microbiological resistance. Still, new ideas and trends are opening the path for safer and more effective answers.
The development of resistant bacteria strains presents one of the most urgent problems in chemical sterilization. Microorganisms change to fit different sterilizing agents, so their survival rate rises and more strong or new agents must be developed. In healthcare environments where sterility is critical, this resistance can cause sterilization failures, so posing major hazards. 2019 Evrova et al.
Another important issue is material compatibility—that is, how well sterilizing agents mix with different materials. Many chemical substances can either destroy or change the characteristics of delicate materials used in packaging and medical equipment. For some polymers, for example, ethylene oxide and formaldehyde can damage structural integrity (Krug, 2023). This can complicate sterilization techniques since it requires careful choice of sterilizing techniques depending on the relevant materials.
Often strict regulatory systems covering chemical sterilization demand thorough validation and documentation to guarantee effectiveness and safety. Following these rules could be resource-intensive and might prevent the acceptance of creative sterilizing technologies (McEVoy & Rowan, 2019). Furthermore delaying the introduction of new sterilizing techniques into clinical practice are the demands for thorough testing and approval procedures.
Recent developments in plasma technology have resulted in the creation of plasma-activated water (PAW), which shows antibacterial effects free of the negative residues connected with conventional chemical agents. Exposing water to plasma generates reactive species that can efficiently kill bacteria (Zhou et al., 2020), hence generating PAW. Food safety and biomedicine are only two of the several disciplines where this approach is attracting interest for possible uses.
Emerging as a good substitute for low-temperature sterilization is vaporized hydrogen peroxide (VH2O2). It breaks down into oxygen and water, so reducing environmental impact and producing no damaging leftovers. This technique has showed promise in overcoming some of the constraints connected with ethylene oxide (McEVoy & Rowan, 2019) and is especially appropriate for the terminal sterilizing of medical equipment. Still, operational difficulties abound, especially for industrial-scale uses (McEVoy & Rowan, 2019).
Methods of Multimodal Sterilization: Physical and chemical sterilizing techniques are starting to be more and more combined in popularity. Combining pulsed electric fields with chemical agents, for instance, can improve antibacterial efficacy while lowering the needed chemical concentration, hence limiting possible material degradation and environmental impact (Chen et al., 2022). Future sterilizing technologies are expected to have great potential in this multimodal area.
Inventive sterilizing tools: Particularly those using non-thermal plasma, the creation of small and effective sterilizing devices is becoming popular. While being user-friendly and flexible enough for many environments, including hospitals and outpatient clinics, these devices can effectively sterilize (Sato et al., 2021). Device design innovations can increase compliance with sterilizing techniques and help to enable more general usage.
Driven by the necessity to handle present issues including microbial resistance, material compatibility, and regulatory compliance, the terrain of chemical sterilization is changing. Along with creative sterilizing tools, emerging technologies—including plasma-activated water and vaporized hydrogen peroxide—offer interesting answers for safer and more effective sterilization methods. Enhancement of the efficacy and application of chemical sterilizing techniques in many different areas depends on ongoing research and development in this field.
References
Arif, A. and Delclos, G. (2011). Association between cleaning-related chemicals and work-related asthma and asthma symptoms among healthcare professionals. Occupational and Environmental Medicine, 69(1), 35-40. https://doi.org/10.1136/oem.2011.064865
Cardoso, J. and Inthurn, A. (2018). Easy and efficient chemical sterilization of the culture medium for in vitro growth of gerbera using chlorine dioxide (clo2). Ornamental Horticulture, 24(3), 218-224. https://doi.org/10.14295/oh.v24i3.1222
Dai, Z., Ronholm, J., Tian, Y., Sethi, B., & Cao, X. (2016). Sterilization techniques for biodegradable scaffolds in tissue engineering applications. Journal of Tissue Engineering, 7, 204173141664881. https://doi.org/10.1177/2041731416648810
Kurose, N. and Aoyagi, Y. (2016). Development of high power, large area, deep ultraviolet light emitting devices using dynamic microplasma excitation (mipe) of algan multiple quantum wells. Electronics and Communications in Japan, 99(7), 3-11. https://doi.org/10.1002/ecj.11768
Mansour, R., Karimizade, A., Enderami, S., Abasi, M., Amiri, F., Jafarirad, A., … & Mellati, A. (2022). The effect of source animal age, decellularization protocol, and sterilization method on bovine acellular dermal matrix as a scaffold for wound healing and skin regeneration. Artificial Organs, 47(2), 302-316. https://doi.org/10.1111/aor.14415
Seif, M., Motawea, I., Shoreibah, E., El-Maghraby, E., & Shalaby, H. (2022). Evaluation of surface characteristic of ti- implant coated with nano-ceramic and chitosan after sterilization by gamma irradiation. Al-Azhar Dental Journal for Girls, 9(1), 29-37. https://doi.org/10.21608/adjg.2021.50250.1322
Garcia-Haro, M., Valdés, C., Vicente-Guijarro, J., Pérez, C., Fabregate, M., Moreno-Nunez, P., … & Andrés, J. (2021). Decontamination of filtering facepiece respirators using a low-temperature-steam–2%-formaldehyde sterilization process during a pandemic: a safe alternative for re-use. Journal of Hospital Infection, 108, 113-119. https://doi.org/10.1016/j.jhin.2020.10.024
Iwamoto, L., Duailibi, M., Iwamoto, G., Oliveira, D., & Duailibi, S. (2021). Evaluation of ethylene oxide, gamma radiation, dry heat and autoclave sterilization processes on extracellular matrix of biomaterial dental scaffolds.. https://doi.org/10.21203/rs.3.rs-834563/v1
Santovito, A., Cervella, P., & Delpero, M. (2013). Chromosomal damage in peripheral blood lymphocytes from nurses occupationally exposed to chemicals. Human & Experimental Toxicology, 33(9), 897-903. https://doi.org/10.1177/0960327113512338
Chen, J., Li, J., Wang, P., Peng, Y., Wang, C., Wang, J., … & Zhang, D. (2022). Utilizing breakdown discharge of self‐powered triboelectric nanogenerator to realize multimodal sterilization. Advanced Sustainable Systems, 7(3). https://doi.org/10.1002/adsu.202200383
Evrova, O., Kellenberger, D., Scalera, C., Calcagni, M., Giovanoli, P., Vogel, V., … & Buschmann, J. (2019). Impact of uv sterilization and short term storage on the in vitro release kinetics and bioactivity of biomolecules from electrospun scaffolds. Scientific Reports, 9(1). https://doi.org/10.1038/s41598-019-51513-1
Krug, N. (2023). Influence of ethylene oxide and gamma irradiation sterilization processes on the properties of poly-l-lactic-acid (plla) materials. Polymers, 15(16), 3461. https://doi.org/10.3390/polym15163461
McEvoy, B. and Rowan, N. (2019). Terminal sterilization of medical devices using vaporized hydrogen peroxide: a review of current methods and emerging opportunities. Journal of Applied Microbiology, 127(5), 1403-1420. https://doi.org/10.1111/jam.14412
Sato, T., Okazaki, K., Nakajima, T., Fujimura, S., & Nakatani, T. (2021). Development of a compact plasma sterilization device for contact lenses. Plasma Medicine, 11(1), 57-67. https://doi.org/10.1615/plasmamed.2021037288
Zhou, R., Zhou, R., Wang, P., Xian, Y., Mai‐Prochnow, A., Lü, X., … & Bazaka, K. (2020). Plasma-activated water: generation, origin of reactive species and biological applications. Journal of Physics D Applied Physics, 53(30), 303001. https://doi.org/10.1088/1361-6463/ab81cf