Xenon: Unique Properties and Expanding Applications
This review investigates the characteristics, isotopic composition, and bonding behavior of xenon, focusing on its numerous applications in lighting, anesthetic, and imaging, as well as growing opportunities in space exploration and environmental technology.
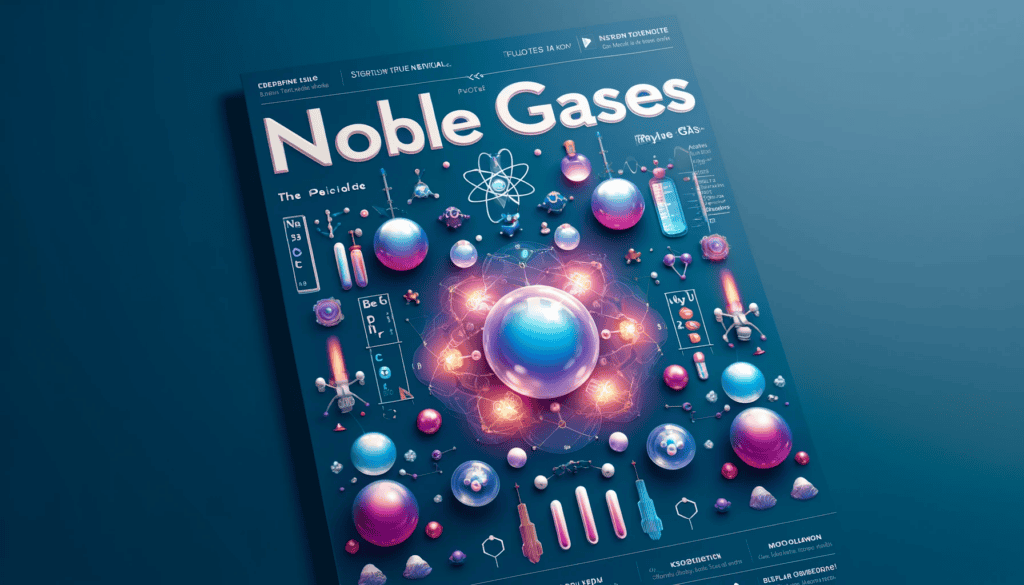
Introduction:
Xenon, a noble gas found in trace levels in the Earth’s atmosphere, has been known to cause global anesthesia since the 1940s (Slupe and Kirsch, 2018). Despite its low abundance in the atmosphere, xenon exhibits distinct isotopic properties, being enriched in heavy isotopes as compared to chondritic or solar xenon, a phenomenon known as the “xenon paradox” (Martin, 2012). This noble gas has demonstrated promising results in scientific studies, particularly in medicine. Xenon has been extensively explored for its neuroprotective characteristics, and it shows great potential as an anesthetic with a low risk profile (Hobbs et al., 2008). Xenon has been shown in studies to provide long-term functional and histopathologic neuroprotection following hypoxia/ischemia, making it a useful agent in such settings (Hobbs et al., 2008).
Xenon has been shown to activate HIF-1α, providing protection against kidney ischemic-reperfusion injury, highlighting its potential applications beyond anesthesia (Ma et al., 2009). Recent research has looked at the molecular pharmacology of xenon, offering light on its modes of action as an anesthetic and neuroprotectant (Dickinson and Franks, 2010). Xenon has shown advantages over other anesthetics in terms of safety, cardiovascular stability, and neuroprotection, making it a useful asset in clinical practice (Sanders et al., 2005).
The historical backdrop surrounding William Ramsay and Morris Travers’ discovery of xenon in 1898 adds to its significance in scientific research (Gonçalves et al., 2022). Despite constraints like as scarcity and production costs, attempts are continuing to build closed xenon delivery systems for greater clinical usage, demonstrating the ongoing interest in exploiting xenon’s characteristics for various purposes (David et al., 2009).
Xenon, being a noble gas with distinct properties and applications, has enormous potential in scientific study, particularly in medicine, anesthesia, and neuroprotection. Its historical significance, together with current advances in understanding its pharmacology and applications, establishes xenon as a key component in scientific research and innovation.
Properties and Characteristics:
Xenon, a noble gas with atomic number 54, has a stable atomic structure with a full valence shell, rendering it innocuous under normal conditions (Bartik et al., 1998). This inertness is due to its high ionization energy and electron affinity, which result in low reactivity with other elements (Peng et al., 2016). Xenon has a number of isotopes, with xenon-129 being the most plentiful and widely investigated due to its advantageous nuclear characteristics for NMR spectroscopy (Moudrakovski et al. 2002). Given xenon’s unwillingness to form chemical bonds, van der Waals forces dominate its bonding behavior. However, at high conditions, xenon may form compounds with elements such as fluorine, oxygen, and carbon, demonstrating its ability to form bonds (Hermann & Schwerdtfeger, 2014; Dewaele et al., 2016; Zhu et al. 2012).
According to Sikora et al. (2012), xenon has a van der Waals radius of about 2 Å, making it extremely polarizable and hydrophobic. This characteristic contributes to xenon’s ability to build inclusion compounds with host molecules, such as cryptophanes, demonstrating its adaptability in a variety of settings (Sikora et al., 2012). Furthermore, xenon’s reactivity in compounds is demonstrated by the creation of xenon oxides and fluorides at high pressures, showing a transition from an inert to a more reactive state (Olia et al. 2009; Dewaele et al. 2016). Studies have also shown that xenon plays a role in stabilizing structures such as metal-organic frameworks and proteins, stressing its influence on the structural characteristics of various materials (Ueda et al., 2007).
Xenon’s atomic structure, physical properties, isotopic composition, bonding behavior, and reactivity in compounds all contribute to its distinct traits and numerous applications in domains ranging from chemistry to biology. Despite being a noble gas, xenon’s capacity to create compounds under certain conditions makes it important in scientific study and technological breakthroughs.
Applications and Future Prospects:
Xenon, known for its many applications, is currently used in a variety of industries, including illumination, anesthetic, and imaging. In lighting, xenon is used in high-intensity discharge lamps because of its ability to create a bright, white light, making it excellent for automobile headlights and high-end film projectors (Babaee et al., 2021). Furthermore, in the medical arena, xenon is prized for its anesthetic qualities, which provide a safe and effective alternative to generating general anesthesia. Its neuroprotective properties have also been investigated, with promising results in circumstances such as hypoxia/ischemia and renal ischemic-reperfusion injury. Additionally, xenon has played a crucial role in imaging technology, particularly magnetic resonance imaging (MRI). Researchers have used hyperpolarized xenon to improve the sensitivity and contrast of MRI, allowing for high-resolution molecular imaging (Schröder et al., 2006; Zhou et al., 2010).
Looking ahead, xenon’s uses in space exploration show potential. According to research, xenon may play an important role in providing safe and effective anesthetic during future space missions, safeguarding astronauts’ well-being in harsh conditions. Furthermore, xenon’s potential for dark matter detection, particularly in detectors based on liquefied noble gas targets such as liquid xenon, offers promising opportunities for furthering our understanding of the cosmos (Aalbers et al., 2016). In terms of medical and environmental technologies, xenon’s developing functions are equally appealing. From cognitive augmentation in neuroscience to the development of focused biosensors for molecular imaging, xenon’s unique features open up new possibilities in healthcare and environmental monitoring (Cinel et al., 2019; Schröder et al., 2006).
In conclusion, xenon’s current applications in lighting, anesthetic, and imaging demonstrate its adaptability and importance in a variety of domains. As we look ahead, the emerging roles of xenon in space exploration, medical technologies, and environmental applications provide exciting opportunities for future research and innovation, highlighting the noble gas’s long-term importance in scientific exploration and technological advancement.
References
David, H., Haelewyn, B., Chazalviel, L., Lecocq, M., Degoulet, M., Risso, J., … & Abraini, J. (2009). Post-ischemic helium provides neuroprotection in rats subjected to middle cerebral artery occlusion-induced ischemia by producing hypothermia. Journal of Cerebral Blood Flow & Metabolism, 29(6), 1159-1165. https://doi.org/10.1038/jcbfm.2009.40
Dickinson, R. and Franks, N. (2010). Bench-to-bedside review: molecular pharmacology and clinical use of inert gases in anesthesia and neuroprotection. Critical Care, 14(4), 229. https://doi.org/10.1186/cc9051
Gonçalves, M., Andolpho, G., Cunha, E., & Ramalho, T. (2022). Exploring 129xe nmr parameters for structural investigation of biomolecules: relativistic, solvent, and thermal effects.. https://doi.org/10.26434/chemrxiv-2022-kmbsn
Hobbs, C., Thoresen, M., Tucker, A., Aquilina, K., Chakkarapani, E., & Dingley, J. (2008). Xenon and hypothermia combine additively, offering long-term functional and histopathologic neuroprotection after neonatal hypoxia/ischemia. Stroke, 39(4), 1307-1313. https://doi.org/10.1161/strokeaha.107.499822
Ma, D., Lim, T., Xu, J., Tang, H., Wan, Y., Zhao, H., … & Maze, M. (2009). Xenon preconditioning protects against renal ischemic-reperfusion injury via hif-1α activation. Journal of the American Society of Nephrology, 20(4), 713-720. https://doi.org/10.1681/asn.2008070712
Marty, B. (2012). The origins and concentrations of water, carbon, nitrogen and noble gases on earth. Earth and Planetary Science Letters, 313-314, 56-66. https://doi.org/10.1016/j.epsl.2011.10.040
Sanders, R., Ma, D., & Maze, M. (2005). Xenon: elemental anaesthesia in clinical practice. British Medical Bulletin, 71(1), 115-135. https://doi.org/10.1093/bmb/ldh034
Slupe, A. and Kirsch, J. (2018). Effects of anesthesia on cerebral blood flow, metabolism, and neuroprotection. Journal of Cerebral Blood Flow & Metabolism, 38(12), 2192-2208. https://doi.org/10.1177/0271678×18789273
Bartik, K., Luhmer, M., Dutasta, J., Collet, A., & Reisse, J. (1998). 129xe and 1h nmr study of the reversible trapping of xenon by cryptophane-a in organic solution. Journal of the American Chemical Society, 120(4), 784-791. https://doi.org/10.1021/ja972377j
Dewaele, A., Worth, N., Pickard, C., Needs, R., Pascarelli, S., Mathon, O., … & Irifune, T. (2016). Synthesis and stability of xenon oxides xe2o5 and xe3o2 under pressure. Nature Chemistry, 8(8), 784-790. https://doi.org/10.1038/nchem.2528
Hermann, A. and Schwerdtfeger, P. (2014). Xenon suboxides stable under pressure. The Journal of Physical Chemistry Letters, 5(24), 4336-4342. https://doi.org/10.1021/jz502230b
Moudrakovski, I., Terskikh, V., Ratcliffe, C., Ripmeester, J., Wang, Y., & Shin, Y. (2002). A 129xe nmr study of functionalized ordered mesoporous silica. The Journal of Physical Chemistry B, 106(23), 5938-5946. https://doi.org/10.1021/jp014585a
Olia, A., Casjens, S., & Cingolani, G. (2009). Structural plasticity of the phage p22 tail needle gp26 probed with xenon gas. Protein Science, 18(3), 537-548. https://doi.org/10.1002/pro.53
Peng, F., Botana, J., Wang, Y., Ma, Y., & Miao, M. (2016). Unexpected trend in stability of xe–f compounds under pressure driven by xe–xe covalent bonds. The Journal of Physical Chemistry Letters, 7(22), 4562-4567. https://doi.org/10.1021/acs.jpclett.6b01922
Sikora, B., Wilmer, C., Greenfield, M., & Snurr, R. (2012). Thermodynamic analysis of xe/kr selectivity in over 137 000 hypothetical metal–organic frameworks. Chemical Science, 3(7), 2217. https://doi.org/10.1039/c2sc01097f
Ueda, T., Kurokawa, K., Eguchi, T., Kachi-Terajima, C., & Takamizawa, S. (2007). Local structure and xenon adsorption behavior of metal−organic framework system [m2(o2cph)4(pyz)]n (m = rh and cu) as studied with use of single-crystal x-ray diffraction, adsorption isotherm, and xenon-129 nmr. The Journal of Physical Chemistry C, 111(3), 1524-1534. https://doi.org/10.1021/jp065321x
Zhu, Q., Jung, D., Oganov, A., Glass, C., Gatti, C., & Lyakhov, A. (2012). Stability of xenon oxides at high pressures. Nature Chemistry, 5(1), 61-65. https://doi.org/10.1038/nchem.1497
Aalbers, J., Agostini, F., Alfonsi, M., Amaro, F., Amsler, C., Aprile, E., … & Zuber, Κ. (2016). Darwin: towards the ultimate dark matter detector. Journal of Cosmology and Astroparticle Physics, 2016(11), 017-017. https://doi.org/10.1088/1475-7516/2016/11/017
Babaee, S., Hashemi, H., & Naidoo, P. (2021). Application of gas hydrates in the separation and purification of xenon from a mixture of xenon and argon. Journal of Chemical & Engineering Data, 66(10), 3815-3825. https://doi.org/10.1021/acs.jced.1c00435
Cinel, C., Valeriani, D., & Poli, R. (2019). Neurotechnologies for human cognitive augmentation: current state of the art and future prospects. Frontiers in Human Neuroscience, 13. https://doi.org/10.3389/fnhum.2019.00013
Komorowski, M., Fleming, S., Mawkin, M., & Hinkelbein, J. (2018). Anaesthesia in austere environments: literature review and considerations for future space exploration missions. NPJ Microgravity, 4(1). https://doi.org/10.1038/s41526-018-0039-y
Schröder, L., Lowery, T., Hilty, C., Wemmer, D., & Pines, A. (2006). Molecular imaging using a targeted magnetic resonance hyperpolarized biosensor. Science, 314(5798), 446-449. https://doi.org/10.1126/science.1131847
Zhou, X., Sun, Y., Mazzanti, M., Henninger, N., Mansour, J., Fisher, M., … & Albert, M. (2010). Mri of stroke using hyperpolarized 129xe. NMR in Biomedicine, 24(2), 170-175. https://doi.org/10.1002/nbm.1568