Click Chemistry: Transforming Synthesis in Modern Science
This study examines click chemistry’s critical role in modern synthetic applications, focusing on recent advances and future directions in medicines, bioconjugation, and material sciences, and emphasizing its transformational impact on chemical synthesis.
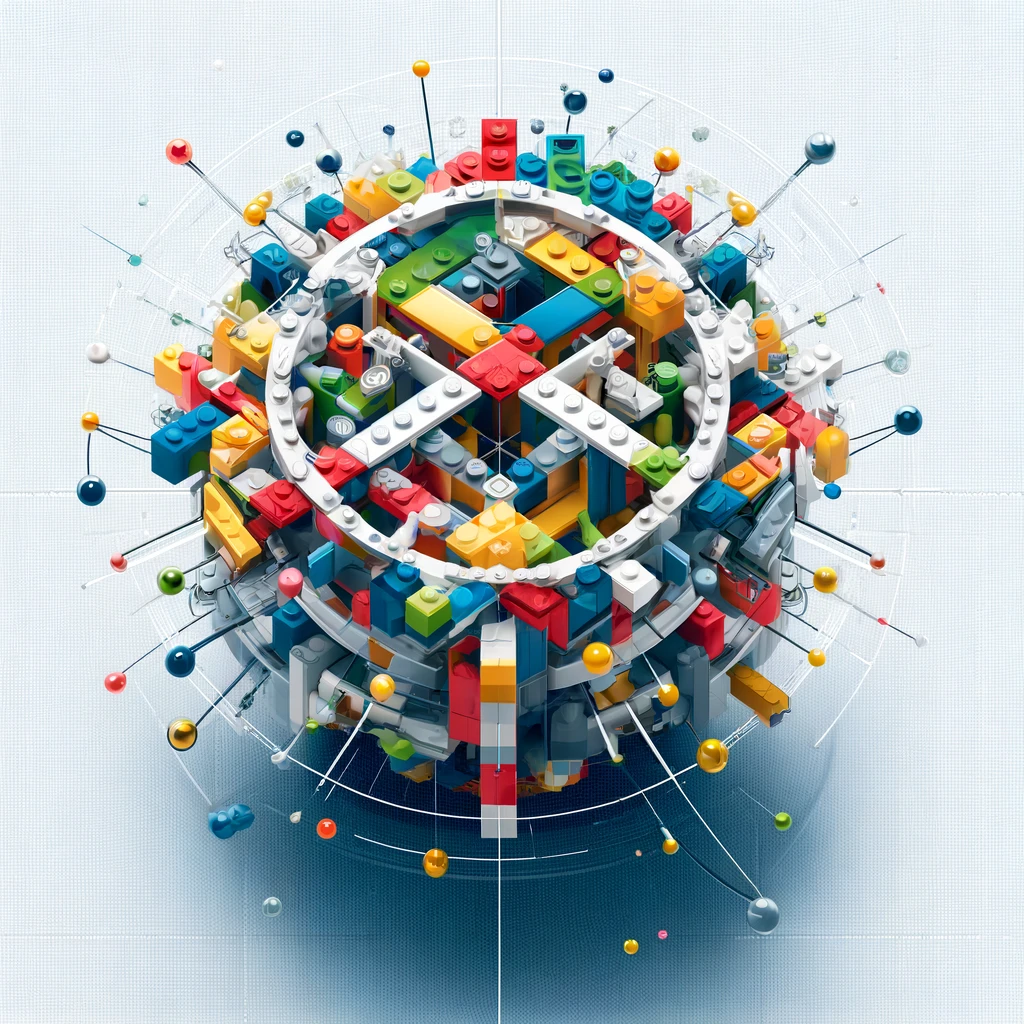
Introduction to Click Chemistry
K. Barry Sharpless and his colleagues introduced click chemistry in 2001, and it has had a substantial impact on synthetic chemistry since it provides very efficient and selective reactions. This method uses a small number of processes renowned for their efficiency and selectivity (Hein et al., 2008). Click chemistry is distinguished by its simplicity, high yields, regiospecificity, and ease of purification, making it a useful tool in a variety of domains including materials science, medicines, and chemical proteomics (Yao et al., 2021). The concept focuses on finding new methods for synthesising carbon-heteroatom bonds, which have numerous applications in chemical proteomics (Dijk et al., 2009).
One of the most important properties of click chemistry is its capacity to assist the synthesis of big macromolecules by efficiently linking tiny building blocks via heteroatom-containing links (Lallana et al., 2011). This has resulted in the development of numerous applications, including medication delivery systems, nanosystems, and the functionalization of various types of nanoparticles (Taşdelen, 2011). Click chemistry has also played a role in the construction of innovative macromolecular structures, addressing the difficulty of coupling polymers produced by various controlled polymerization processes (Kamphuis et al., 2010).
Click chemistry is significant because of its wide range of applications and the ease with which complex compounds and materials can be synthesized from modular blocks (Kade et al., 2010). This technique has been extended beyond metal-catalyzed cycloaddition reactions, prompting researchers to investigate alternative chemical processes in many domains of chemistry (Becer et al., 2009). Because of its simplicity and reliability, the concept of click chemistry has sparked great interest in a variety of areas, including materials science, polymer chemistry, and biology (Fournier et al., 2007).
K. Barry Sharpless pioneered click chemistry, which has emerged as a strong tool in synthetic chemistry, providing efficient, selective, and high-yielding reactions that have transformed the way complex compounds and materials are manufactured across multiple scientific areas.
Applications of Click Chemistry
Because of its adaptability and significance, click chemistry is widely used in a variety of domains, including bioconjugation, medicines, and materials research. In bioconjugation, the combination of expressed protein ligation (EPL) with click chemistry has led to the development of highly efficient and site-specific bioconjugation processes, assuring uniform orientation of targeted ligands. Elias, et al. (2013).
In pharmaceuticals, click chemistry has been used to create anticancer, antimicrobial, and biomedical imaging drugs. Cu-catalyzed click chemistry (CuAAC) has received special attention for its extensive applicability and efficiency in pharmaceutical sciences (Kaur et al., 2021; Tiwari et al., 2016). Click chemistry has also helped to produce new therapies, improve bioconjugation efficiency, and facilitate the creation of combinatorial libraries for drug discovery (Pokorski & Smith, 2018).
In material science, click chemistry has proved critical in functionalizing nanoparticles for a variety of purposes. For example, using click chemistry for quantum dot (QD) functionalization shows promise for generating bioconjugates for in vivo applications (Beaune et al., 2011). Click chemistry has also been used to produce and functionalize polymers, dendrimers, and hydrogels for drug delivery systems, demonstrating its importance in material science (Lallana et al. 2012).
Click chemistry has had a significant impact on bioconjugation, pharmaceuticals, and material science by allowing for efficient and varied reactions that have aided the development of tailored bioconjugates, innovative pharmacological agents, and functionalized materials for a variety of uses.
Recent Advances and Future Directions
Recent breakthroughs in click chemistry have resulted in tremendous progress in the creation of biorthogonal reactions, broadening its applications across a variety of sectors. Biorthogonal reactions, such as strain-promoted azide-alkyne cycloaddition (SPAAC) and inverse electron-demand Diels-Alder (IEDDA) reactions, have gained prominence due to their bioorthogonal nature, allowing for specific and selective labeling of biomolecules in complex biological systems (Battigelli et al., 2022; Simeonova & Todorov, 2023).
In the field of environmental sustainability, click chemistry has shown promise in the creation of bioorthogonal processes for tissue engineering and medication delivery systems. For example, using biocompatible materials and biorthogonal click chemistry has resulted in the development of porous organic-inorganic nanohybrids for bone repair that mirror native bone tissue architectures (Liu et al., 2023). Furthermore, the use of click chemistry in extracellular vesicles (EVs) for targeted medication administration demonstrates its potential in environmentally friendly delivery methods (Ciferri, 2024).
In medical diagnostics, recent advances in click chemistry have resulted in the development of genetically encoded click reactions for spatially constrained metabolite labeling, allowing for precise labeling of biomolecules in living cell systems (Mishra et al., 2023). Furthermore, the use of click chemistry in the production of contrast agents for magnetic resonance imaging (MRI) has improved imaging performance and paved the way for future advances in diagnostic imaging techniques (Hapuarachchige & Artemov, 2016).
Future click chemistry developments are expected to focus on developing bioorthogonal processes for more complicated biological applications, such as tissue engineering and drug delivery systems. Continued research on click chemistry in environmental sustainability, particularly the development of biocompatible materials and targeted delivery techniques, is likely to stimulate innovation in green chemical practices. Furthermore, the incorporation of click chemistry into medical diagnostics is expected to change imaging procedures and improve diagnostic accuracy in a variety of healthcare settings.
References
Becer, C., Hoogenboom, R., & Schubert, U. (2009). Click chemistry beyond metal‐catalyzed cycloaddition. Angewandte Chemie, 48(27), 4900-4908. https://doi.org/10.1002/anie.200900755
Dijk, M., Rijkers, D., Liskamp, R., Nostrum, C., & Hennink, W. (2009). Synthesis and applications of biomedical and pharmaceutical polymers via click chemistry methodologies. Bioconjugate Chemistry, 20(11), 2001-2016. https://doi.org/10.1021/bc900087a
Fournier, D., Hoogenboom, R., & Schubert, U. (2007). Clicking polymers: a straightforward approach to novel macromolecular architectures. Chemical Society Reviews, 36(8), 1369. https://doi.org/10.1039/b700809k
Hein, C., Liu, X., & Wang, D. (2008). Click chemistry, a powerful tool for pharmaceutical sciences. Pharmaceutical Research, 25(10), 2216-2230. https://doi.org/10.1007/s11095-008-9616-1
Kade, M., Burke, D., & Hawker, C. (2010). The power of thiol‐ene chemistry. Journal of Polymer Science Part a Polymer Chemistry, 48(4), 743-750. https://doi.org/10.1002/pola.23824
Kamphuis, M., Johnston, A., Such, G., Dam, H., Evans, R., Scott, A., … & Caruso, F. (2010). Targeting of cancer cells using click-functionalized polymer capsules. Journal of the American Chemical Society, 132(45), 15881-15883. https://doi.org/10.1021/ja106405c
Lallana, E., Sousa-Hervés, A., Fernández-Trillo, F., Riguera, R., & Fernández-Megía, E. (2011). Click chemistry for drug delivery nanosystems. Pharmaceutical Research, 29(1), 1-34. https://doi.org/10.1007/s11095-011-0568-5
Lutz, J. (2007). 1,3‐dipolar cycloadditions of azides and alkynes: a universal ligation tool in polymer and materials science. Angewandte Chemie, 46(7), 1018-1025. https://doi.org/10.1002/anie.200604050
Taşdelen, M. (2011). Diels–alder “click” reactions: recent applications in polymer and material science. Polymer Chemistry, 2(10), 2133. https://doi.org/10.1039/c1py00041a
Yao, T., Xu, X., & Huang, R. (2021). Recent advances about the applications of click reaction in chemical proteomics. Molecules, 26(17), 5368. https://doi.org/10.3390/molecules26175368
Beaune, G., Tamang, S., Bernardin, A., Bayle–Guillemaud, P., Fenel, D., Schoehn, G., … & Texier, I. (2011). Luminescence of polyethylene glycol coated cdsete/zns and inp/zns nanoparticles in the presence of copper cations. Chemphyschem, 12(12), 2247-2254. https://doi.org/10.1002/cphc.201100266
Elias, D., Poloukhtine, A., Popik, V., & Tsourkas, A. (2013). Effect of ligand density, receptor density, and nanoparticle size on cell targeting. Nanomedicine Nanotechnology Biology and Medicine, 9(2), 194-201. https://doi.org/10.1016/j.nano.2012.05.015
Kaur, J., Saxena, M., & Rishi, N. (2021). An overview of recent advances in biomedical applications of click chemistry. Bioconjugate Chemistry, 32(8), 1455-1471. https://doi.org/10.1021/acs.bioconjchem.1c00247
Lallana, E., Fernández-Trillo, F., Sousa-Hervés, A., Riguera, R., & Fernández-Megía, E. (2012). Click chemistry with polymers, dendrimers, and hydrogels for drug delivery. Pharmaceutical Research, 29(4), 902-921. https://doi.org/10.1007/s11095-012-0683-y
Pokorski, J. and Smith, L. (2018). “click” chemistry for medicine and biology. Molecular Pharmaceutics, 15(8), 2891-2891. https://doi.org/10.1021/acs.molpharmaceut.8b00743
Tiwari, V., Mishra, B., Mishra, K., Mishra, N., Singh, A., & Chen, X. (2016). Cu-catalyzed click reaction in carbohydrate chemistry. Chemical Reviews, 116(5), 3086-3240. https://doi.org/10.1021/acs.chemrev.5b00408
Battigelli, A., Almeida, B., & Shukla, A. (2022). Recent advances in bioorthogonal click chemistry for biomedical applications. Bioconjugate Chemistry, 33(2), 263-271. https://doi.org/10.1021/acs.bioconjchem.1c00564
Ciferri, M. (2024). Standardized method to functionalize plasma-extracellular vesicles via copper-free click chemistry for targeted drug delivery strategies. Acs Applied Bio Materials, 7(2), 827-838. https://doi.org/10.1021/acsabm.3c00822
Hapuarachchige, S. and Artemov, D. (2016). Click chemistry in the development of contrast agents for magnetic resonance imaging. Topics in Magnetic Resonance Imaging, 25(5), 205-213. https://doi.org/10.1097/rmr.0000000000000099
Liu, X., Gaihre, B., Li, L., Rezaei, A., Tilton, M., Elder, B., … & Lu, L. (2023). Bioorthogonal “click chemistry” bone cement with bioinspired natural mimicking microstructures for bone repair. Acs Biomaterials Science & Engineering, 9(3), 1585-1597. https://doi.org/10.1021/acsbiomaterials.2c01482
Mishra, P., Sharma, N., Kim, H., Lee, C., & Rhee, H. (2023). Gen-click: genetically encodable click reactions for spatially restricted metabolite labeling. Acs Central Science, 9(8), 1650-1657. https://doi.org/10.1021/acscentsci.3c00511
Simeonova, G. and Todorov, B. (2023). Modification of [18f]fdg by the formation of a hydrazone bond. Journal of Imab – Annual Proceeding (Scientific Papers), 29(1), 4784-4788. https://doi.org/10.5272/jimab.2023291.4784