Next-Gen Biomaterials for Medical Innovations
This review looks at the transformative influence of next-generation bioconjugate biomaterials on medicine. It looks into their applications in hydrogel enhancement, biomolecule stabilization, and biological process knowledge, emphasizing their great potential for disease detection, therapy, and human health insights.
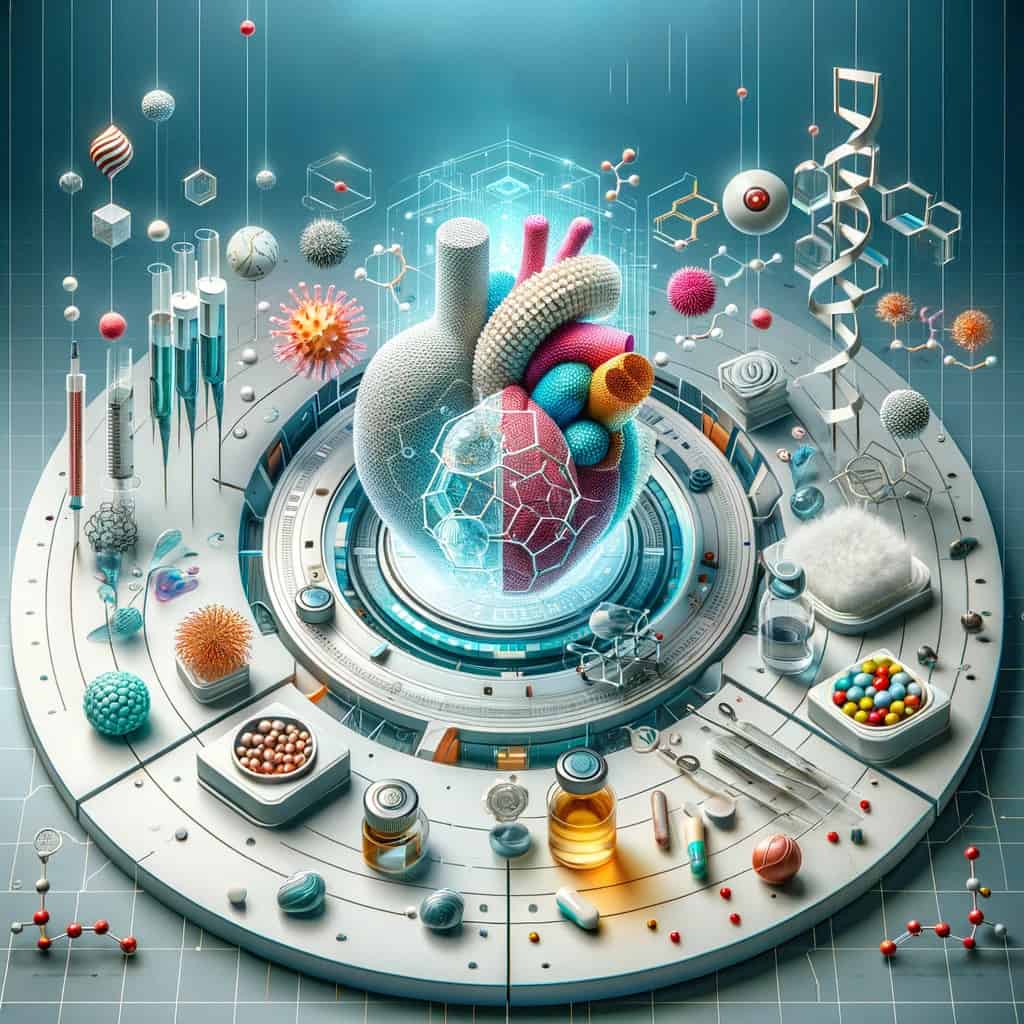
1. Introduction to Bioconjugate Biomaterials
Bioconjugate biomaterials have emerged as a key field in modern medicine, offering novel approaches to disease diagnosis and treatment. These biomaterials are critical for improving hydrogels and stabilizing proteins and peptides, hence altering a variety of medical applications (Ahadian et al., 2015). Bioconjugation techniques allow researchers to covalently connect various compounds to generate functional combinations of biomolecules customized for specific biological applications (Ahadian et al., 2015). Efficient coupling chemistries found in recent decades have made it possible to create innovative nanometer- and micron-sized structures made of polymer-peptide bioconjugates.
Gold nanoparticles (AuNPs) have emerged as intriguing candidates for the development of novel biomaterials, with unique features for researching biological systems (Yeh et al. 2012). Furthermore, the use of polyglycerol as a multifunctional polyether in bioconjugation has shown promise for applications in degradable biomaterials and diagnostics, establishing polyglycerol as a promising alternative to poly(ethylene glycol) (PEG) (Thomas et al., 2014).
Bioconjugated hydrogels have showed great promise in tissue engineering and regenerative medicine applications. These hydrogels, made via bioconjugation processes, have specialized functions for a variety of biomedical applications, including tissue scaffolds and microfluidics (Ahadian et al. 2015). Furthermore, the development of cardiac patches employing smart biomaterials demonstrates the potential of bioconjugates to drive new concepts in biocytology and medicine, providing promising treatments for disorders such as myocardial infarction (Zhang et al., 2022).
The subject of bioconjugation is constantly evolving, with the introduction of novel approaches and capacities. Site-specific bioconjugation techniques are critical for maintaining protein structure and function, assuring the success of bioconjugate applications in a variety of disciplines such as biologic drug development and biomaterial synthesis (Ohata et al., 2018). Furthermore, incorporating extracellular matrix-derived structures into soft biomaterials improves cell adhesion and proliferation, highlighting bioconjugates’ versatility and promise for tissue regeneration (Kascholke et al., 2017).
Bioconjugate biomaterials represent a new frontier in medical innovation, providing tailored solutions for illness diagnostics, treatment, and tissue engineering. Researchers are paving the road for improved biomedical applications by utilizing bioconjugation techniques and leveraging the unique features of materials like gold nanoparticles and polyglycerol. The continued development of site-specific bioconjugation technologies, as well as the incorporation of bioconjugates into biomaterials, show enormous promise for the future of modern medicine.
2. Engineering and Applications
Bioconjugate biomaterials have had a substantial impact on tissue engineering and regenerative medicine by providing tailored solutions for a wide range of applications, including stem cell signaling, cellular mobility, and tissue healing. These bioconjugate biomaterials have shown promise for influencing immune responses, facilitating tissue regeneration, and improving treatment outcomes (Hall et al., 2016).
One significant application of bioconjugate biomaterials is to promote soft tissue regeneration in the musculoskeletal system by altering macrophage polarization (Ye et al., 2021). The mechanical properties of these biomaterials are critical in tissue engineering, notably tendon and ligament repair. Furthermore, bioconjugate biomaterials have played an important role in bone regeneration, promoting tissue engineering for future application as biological substitutes to heal or regenerate injured tissues (Zhu et al., 2021).
In the field of cardiac tissue engineering, bioconjugated silk-based biomaterials have showed potential in improving cardiac repair techniques. Furthermore, the creation and application of biomimetic self-assembled peptide hydrogels has made tremendous progress in hard tissue regeneration, particularly bone and dental tissue engineering (Najafi et al., 2021). Furthermore, the use of plant-derived biomaterials in cardiac tissue engineering demonstrates the wide potential of bioconjugates in regenerative medicine (Dai et al., 2023).
Bioconjugate biomaterials have a significant impact on human health, particularly immunomodulatory biomaterials that play an important role in tissue repair and regeneration (Whitaker et al., 2021). These biomaterials have the ability to regulate immune responses, speed up wound healing, and promote tissue regeneration. Furthermore, advances in bone healing biomaterials represent a transition from bioinert to bioactive materials, ushering in a new era of tissue engineering (Wang et al., 2022).
Looking ahead, the development of reactive oxygen species-based biomaterials for regenerative medicine and tissue engineering applications shows great promise (Shafiq et al., 2021). Novel immunomodulation techniques in tissue restoration are being investigated by exploiting biomaterial interactions with the immunological milieu (Pinho et al., 2021). Furthermore, the use of electroactive smart materials for neural tissue regeneration exemplifies the novel methodologies being pursued in biomaterials engineering (Liu et al., 2022).
Bioconjugate biomaterials have created new opportunities in tissue engineering and regenerative medicine by providing personalized solutions for boosting tissue repair, understanding stem cell activity, and improving therapeutic treatments. The ongoing advancement of biomaterial design and applications is poised to revolutionize the landscape of modern healthcare, paving the path for novel treatments and better patient outcomes.
3. Challenges and Future Directions
Current challenges in developing and applying next-generation active materials:
The research and implementation of next-generation active materials encounter a number of hurdles that must be overcome in order to progress further. One key problem is to precisely control and manipulate the features of these materials, such as their mechanical, electrical, and chemical characteristics, to fulfill the specific requirements of varied biological applications. Tu, et al. (2014). Achieving a balance between material functionality and biocompatibility remains a significant problem, particularly when creating materials for implanted devices and medication delivery systems (Khan, 2024). Furthermore, maintaining the long-term stability and safety of these materials in biological contexts is a challenge that necessitates extensive research and optimization (Kratz et al. 2012).
Another challenge is to improve our understanding of the interactions between active materials and biological systems so that we can effectively predict and manage their behavior. This includes investigating the cellular absorption methods, biodegradation pathways, and potential toxicity of these compounds to ensure their safe and effective use in biomedical applications (Kuang, 2023). Furthermore, the scalability and cost-effectiveness of production techniques for next-generation active materials pose issues that must be addressed before they can be widely adopted in healthcare settings (Zhang et al., 2023).
Future Research Directions and Possible Breakthroughs:
To solve current obstacles and foster innovation in the field of next-generation active materials, future research should concentrate on a few important areas. One interesting direction is the development of stimulus-responsive materials, which may adjust their characteristics in response to specific biological cues or external stimuli, allowing for precise control over their behavior in complicated biological contexts (Mendiratta et al., 2019). Researchers can fully realize the potential of these materials for drug delivery, tissue engineering, and biosensing by investigating novel synthesis methodologies and functionalization strategies (Salama & Hesemann, 2020).
Furthermore, the combination of advanced characterization techniques, such as advanced imaging modalities and spectroscopic methods, can provide valuable insights into the structure-property relationships of active materials at the nanoscale, allowing for the design of materials with tailored functionalities for specific biomedical applications. Harnessing the unique features of two-dimensional materials and nanocomposites holds great promise for generating novel solutions in fields such as cancer therapy, tissue regeneration, and biosensing.
Furthermore, research into bioinspired design principles and biomimetic techniques can inspire the creation of active materials that imitate the sophisticated functions and structures found in nature. Researchers can use biological systems to generate materials with improved biocompatibility, self-healing capabilities, and adaptive responses, leading to breakthroughs in regenerative medicine and customized healthcare.
The Continuous Need for Innovative Materials in Advancing Biomedical Science:
To summarize, the continued need for new materials in biomedical science is clear, as these materials play a critical role in enabling groundbreaking discoveries and transformative healthcare technology. Researchers can fully realize the potential of next-generation active materials for a variety of biomedical applications by tackling current challenges and pursuing new research avenues. The discipline of biomaterials engineering has the potential to create significant advances in healthcare and enhance patient outcomes through interdisciplinary collaboration, cutting-edge research, and a commitment to translational science.
References:
Ahadian, S., Sadeghian, R., Salehi, S., Ostrovidov, S., Bae, H., & Ramalingam, M. (2015). Bioconjugated hydrogels for tissue engineering and regenerative medicine. Bioconjugate Chemistry, 26(10), 1984-2001. https://doi.org/10.1021/acs.bioconjchem.5b00360
Dai, Y., Qiao, K., Li, D., Isingizwe, P., Liu, H., Liu, Y., … & Cui, X. (2023). Plant‐derived biomaterials and their potential in cardiac tissue repair. Advanced Healthcare Materials, 12(20). https://doi.org/10.1002/adhm.202202827
Hall, M., Estrellas, K., Allen, B., Wolf, M., Fan, H., Tam, A., … & Elisseeff, J. (2016). Developing a pro-regenerative biomaterial scaffold microenvironment requires t helper 2 cells. Science, 352(6283), 366-370. https://doi.org/10.1126/science.aad9272
Kascholke, C., Loth, T., Kohn-Polster, C., Möller, S., Bellstedt, P., Schulz‐Siegmund, M., … & Hacker, M. (2017). Dual-functional hydrazide-reactive and anhydride-containing oligomeric hydrogel building blocks. Biomacromolecules, 18(3), 683-694. https://doi.org/10.1021/acs.biomac.6b01355
Khan, A. (2024). Biogenic synthesis of silver nanoparticles using rubus fruticosus extract and their antibacterial efficacy against erwinia caratovora and ralstonia solanacearum phytopathogens. RSC Advances, 14(9), 5754-5763. https://doi.org/10.1039/d3ra06723h
Kratz, K., Voigt, U., & Lendlein, A. (2012). Temperature‐memory effect of copolyesterurethanes and their application potential in minimally invasive medical technologies. Advanced Functional Materials, 22(14), 3057-3065. https://doi.org/10.1002/adfm.201200211
Kuang, F. (2023). Post‐graphene 2d materials: structures, properties, and cancer therapy applications. Advanced Healthcare Materials, 13(5). https://doi.org/10.1002/adhm.202302604
Liu, G., Chen, J., Wang, X., Liu, Y., Ma, Y., & Tu, X. (2022). Functionalized 3d-printed st2/gelatin methacryloyl/polcaprolactone scaffolds for enhancing bone regeneration with vascularization. International Journal of Molecular Sciences, 23(15), 8347. https://doi.org/10.3390/ijms23158347
Luo, T. and Kiick, K. (2017). Collagen-like peptide bioconjugates. Bioconjugate Chemistry, 28(3), 816-827. https://doi.org/10.1021/acs.bioconjchem.6b00673
Mendiratta, S., Hussein, M., Nasser, H., & Ali, A. (2019). Multidisciplinary role of mesoporous silica nanoparticles in brain regeneration and cancers: from crossing the blood–brain barrier to treatment. Particle & Particle Systems Characterization, 36(9). https://doi.org/10.1002/ppsc.201900195
Najafi, H., Jafari, M., Farahavar, G., Abolmaali, S., Azarpira, N., Borandeh, S., … & Ravanfar, R. (2021). Recent advances in design and applications of biomimetic self-assembled peptide hydrogels for hard tissue regeneration. Bio-Design and Manufacturing, 4(4), 735-756. https://doi.org/10.1007/s42242-021-00149-0
Ohata, J., Miller, M., Mountain, C., Vohidov, F., & Ball, Z. (2018). A three‐component organometallic tyrosine bioconjugation. Angewandte Chemie, 57(11), 2827-2830. https://doi.org/10.1002/anie.201711868
Pinho, T., Cunha, C., Lanceros-Méndez, S., & Salgado, A. (2021). Electroactive smart materials for neural tissue regeneration. Acs Applied Bio Materials, 4(9), 6604-6618. https://doi.org/10.1021/acsabm.1c00567
Salama, A. and Hesemann, P. (2020). Recent trends in elaboration, processing, and derivatization of cellulosic materials using ionic liquids. Acs Sustainable Chemistry & Engineering, 8(49), 17893-17907. https://doi.org/10.1021/acssuschemeng.0c06913
Shafiq, M., Chen, Y., Hashim, R., He, C., Mo, X., & Zhou, X. (2021). Reactive oxygen species-based biomaterials for regenerative medicine and tissue engineering applications. Frontiers in Bioengineering and Biotechnology, 9. https://doi.org/10.3389/fbioe.2021.821288
Song, Y., Wang, H., Yue, F., Lv, Q., Cai, B., Dong, N., … & Wang, L. (2020). Silk‐based biomaterials for cardiac tissue engineering. Advanced Healthcare Materials, 9(23). https://doi.org/10.1002/adhm.202000735
Thomas, A., Müller, S., & Frey, H. (2014). Beyond poly(ethylene glycol): linear polyglycerol as a multifunctional polyether for biomedical and pharmaceutical applications. Biomacromolecules, 15(6), 1935-1954. https://doi.org/10.1021/bm5002608
Tu, Q., Pang, L., Chen, Y., Zhang, Y., Zhang, R., Lu, B., … & Wang, J. (2014). Effects of surface charges of graphene oxide on neuronal outgrowth and branching. The Analyst, 139(1), 105-115. https://doi.org/10.1039/c3an01796f
Wang, Y., Zhang, H., Yan, H., Jing, Y., Zhang, G., & Su, J. (2022). Bone repair biomaterials: a perspective from immunomodulation. Advanced Functional Materials, 32(51). https://doi.org/10.1002/adfm.202208639
Whitaker, R., Hernaez-Estrada, B., Hernández, R., Santos‐Vizcaíno, E., & Spiller, K. (2021). Immunomodulatory biomaterials for tissue repair. Chemical Reviews, 121(18), 11305-11335. https://doi.org/10.1021/acs.chemrev.0c00895
Ye, J., Xie, C., Wang, C., Huang, J., Yin, Z., Heng, B., … & Shen, W. (2021). Promoting musculoskeletal system soft tissue regeneration by biomaterial-mediated modulation of macrophage polarization. Bioactive Materials, 6(11), 4096-4109. https://doi.org/10.1016/j.bioactmat.2021.04.017
Yeh, Y., Creran, B., & Rotello, V. (2012). Gold nanoparticles: preparation, properties, and applications in bionanotechnology. Nanoscale, 4(6), 1871-1880. https://doi.org/10.1039/c1nr11188d
Zhang, R., Jiang, J., & Wu, W. (2023). Wearable chemical sensors based on 2d materials for healthcare applications. Nanoscale, 15(7), 3079-3105. https://doi.org/10.1039/d2nr05447g
Zhang, Y., Mu, W., Zhang, Y., He, X., Wang, Y., Ma, H., … & Li, H. (2022). Recent advances in cardiac patches: materials, preparations, and properties. Acs Biomaterials Science & Engineering, 8(9), 3659-3675. https://doi.org/10.1021/acsbiomaterials.2c00348
Zhu, Y., Goh, C., & Shrestha, A. (2021). Biomaterial properties modulating bone regeneration. Macromolecular Bioscience, 21(4). https://doi.org/10.1002/mabi.202000365