Chemical Innovations Shaping the Future of Wearables
This paper examines the critical role of chemistry in the evolution of wearable technology, highlighting recent advances and potential future paths. It digs into recent chemical innovations that have considerably improved the functioning, durability, and user comfort of wearables, with an emphasis on breakthrough materials, sophisticated sensor technologies, and efficient energy storage solutions. The topic expands to the difficulties of biocompatibility, environmental sustainability, and scalability, underlining the importance of interdisciplinary research. Through this examination, we highlight the crucial role of chemical sciences in pushing the limits of wearable devices, providing insights into their disruptive potential in health monitoring, personal electronics, and beyond.
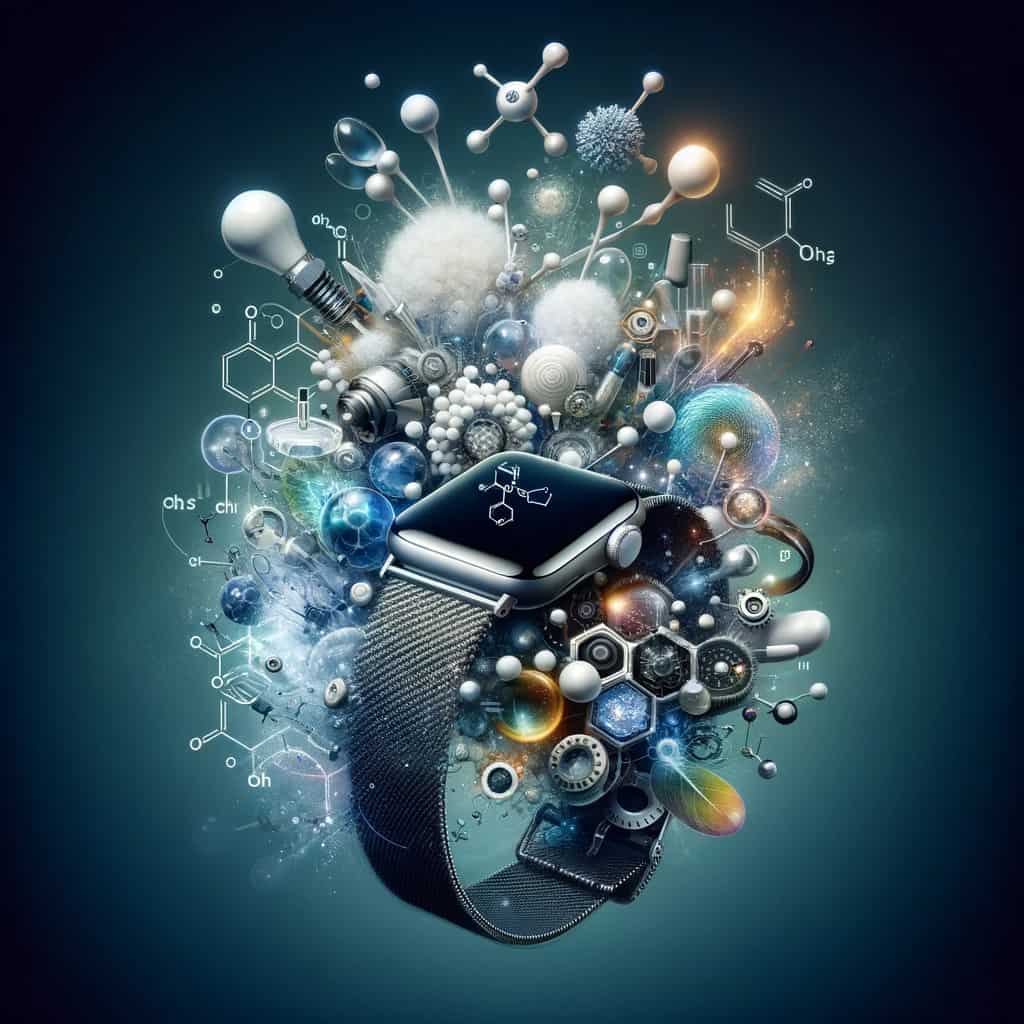
Introduction to Wearable Chemistry
The confluence of chemistry and electronics has led to substantial advancements in wearable devices. Wearables are developed using revolutionary material formulations and technologies that improve features such as surface area accessibility and ion transport within electrodes while taking up minimal space (Yang et al., 2019). For example, the use of paper in wearable electronics has features such as breathability, flexibility, biocompatibility, and biodegradability, as well as customizable surface chemistry and porous architectures. This demonstrates how materials with precise chemical properties might enable the development of functional and comfortable wearable gadgets.
The incorporation of novel polymer chemistries into electronics has resulted in the creation of recyclable, biodegradable, and biocompatible electronic devices (Chiong et al., 2021). This emphasizes the relevance of material chemistry in guaranteeing long-term viability and biological compatibility. Furthermore, substantial study has been undertaken on the role of water in mediating interfacial adhesion and shear strength in materials such as graphene oxide for use in wearable electronics (Soler-Crespo et al. 2018). Understanding chemical interactions is critical for improving the mechanical properties of wearable materials.
Wearable electronics has also made strides in designing flexible hybrid sensors for health monitoring, emphasizing the importance of materials and mechanisms that improve wearability (Gao et al., 2019). Furthermore, the use of sophisticated materials such as MXenes in textile sensors for wearable applications indicates how chemistry is critical to the performance of these devices (Jin & Bai, 2022). These sensors highlight the significance of material design and chemistry in developing wearable electronics that blend effortlessly into everyday life.
Furthermore, the development of carbon-based flexible devices for health monitoring highlights the importance of carbon materials in enabling the fabrication of wearable and flexible devices with a variety of functions and superior performance (Wang et al., 2023). This demonstrates how the use of materials with certain chemical properties can improve the functionality and usability of wearable electronics.
Materials in Wearables
Materials are critical to the development of wearable technologies. Conductive polymers, such as carbon nanotubes (CNTs), graphene-based materials, and carbon black (CB), are widely used in wearable sensors due to their high electrical conductivity, low cost, mass production potential, high chemical and thermal stability, flexibility, and ease of chemical functionalization (Heo et al., 2020). These qualities make conductive polymers excellent for developing sensors that integrate smoothly into wearable devices while remaining useful and comfortable.
Flexible electronics, such as gold nanowires, carbon nanotube electronics, and organic light-emitting diodes, present prospects for creating wearable health monitoring devices with improved wearability and comfort (Koo et al., 2017). These materials are flexible and adaptable to the human body’s movements, allowing wearables to be worn comfortably for long periods of time without discomfort or impeding natural motions.
Energy storage materials, such as MXene-based textiles and porous nanocomposite fabrics, are critical for fueling wearable devices and maintaining continuous operation. MXene materials are known for their excellent conductivity, processability, and mechanical stability, making them ideal for energy storage applications in wearables. Furthermore, the utilization of biodegradable and recyclable biomaterials in energy harvesting devices highlights the significance of sustainability in wearable technology development (Bai et al., 2020).
These materials’ chemical characteristics play a crucial role in their viability as wearables. Conductive polymers have strong electrical conductivity, which is critical for signal transmission and processing in wearable sensors (Harito et al., 2020). Flexible electronics include mechanical flexibility and waterproofing, allowing wearables to survive a variety of weather conditions and movements while remaining functional (Lee et al., 2019). Energy storage materials are highly conductive and mechanically resilient, allowing for efficient energy harvesting and storage for extended wearable device operation (Bai et al., 2020).
Applications and Future Directions
Wearable technology applications such as health monitoring, fitness tracking, and smart textiles have altered the healthcare and wellness industries. Wearable sensors monitor vital signs, physical activity, and environmental conditions, allowing for tailored health management and early detection of health problems. For example, flexible wearable sensors have been designed to monitor cardiovascular health, providing real-time data on vital signs even during movement or harsh situations. Chen, et al. (2021). These sensors provide non-invasive and continual monitoring for individuals, improving the quality of healthcare services.
Wearable gadgets with sensors and actuators allow users to track their physical activity, sleep patterns, and overall fitness levels. These gadgets offer useful insights into everyday activities, enabling users to have a healthy lifestyle and track their progress toward exercise goals (Lyu et al., 2021). Furthermore, smart textiles combined with wearable electronics provide novel options for health monitoring and performance improvement. These textiles may detect physiological signals such as sweat composition and offer users with real-time feedback, resulting in improved health management and performance optimization (Gu et al., 2019).
Furthermore, advances in wearable biosensors have transformed the field of remote healthcare monitoring. Wearable biosensors, particularly those based on microfluidics and additive manufacturing, can detect a variety of biomarkers on the skin, allowing for continuous health monitoring and early disease identification (Padash et al., 2020). These biosensors have the potential to alter healthcare delivery by allowing for more tailored and proactive health management measures.
Looking ahead, wearable chemistry provides enormous potential for bio-integration and self-healing materials in wearable technologies. Bio-integration is the seamless integration of wearable technology with the human body, allowing for comfortable and noninvasive health monitoring. Self-healing materials, on the other hand, can fix damage on their own, increasing the lifespan and functioning of wearable devices (Dong et al., 2020). Researchers hope to improve user experience, boost long-term wearability, and ensure the durability of wearable devices by combining bio-integration and self-healing properties.
To summarize, the present uses of wearable technology in health monitoring, fitness tracking, and smart textiles have had a tremendous impact on healthcare and wellness. Future improvements in wearable chemistry, with a focus on bio-integration and self-healing materials, have the potential to significantly improve the usefulness, comfort, and sustainability of wearable devices, ultimately improving users’ lives.
Reference:
Bai, Z., Xu, Y., Li, J., Zhu, J., Gao, C., Zhang, Y., … & Guo, J. (2020). An eco-friendly porous nanocomposite fabric-based triboelectric nanogenerator for efficient energy harvesting and motion sensing. Acs Applied Materials & Interfaces, 12(38), 42880-42890. https://doi.org/10.1021/acsami.0c12709
Chen, S., Qi, J., Fan, S., Qiao, Z., Yeo, J., & Lim, C. (2021). Flexible wearable sensors for cardiovascular health monitoring. Advanced Healthcare Materials, 10(17). https://doi.org/10.1002/adhm.202100116
Chiong, J., Tran, H., Lin, Y., Zheng, Y., & Bao, Z. (2021). Integrating emerging polymer chemistries for the advancement of recyclable, biodegradable, and biocompatible electronics. Advanced Science, 8(14). https://doi.org/10.1002/advs.202101233
Dong, M., Fang, B., Li, J., Sun, F., & Liu, H. (2020). Wearable sensing devices for upper limbs: a systematic review. Proceedings of the Institution of Mechanical Engineers Part H Journal of Engineering in Medicine, 235(1), 117-130. https://doi.org/10.1177/0954411920953031
Gao, Y., Yu, L., Yeo, J., & Lim, C. (2019). Flexible hybrid sensors for health monitoring: materials and mechanisms to render wearability. Advanced Materials, 32(15). https://doi.org/10.1002/adma.201902133
Gu, Y., Zhang, T., Chen, H., Feng, W., Pu, Y., & Gao, C. (2019). Mini review on flexible and wearable electronics for monitoring human health information. Nanoscale Research Letters, 14(1). https://doi.org/10.1186/s11671-019-3084-x
Harito, C., Utari, L., Putra, B., Yuliarto, B., Purwanto, S., Zaidi, S., … & Walsh, F. (2020). Review—the development of wearable polymer-based sensors: perspectives. Journal of the Electrochemical Society, 167(3), 037566. https://doi.org/10.1149/1945-7111/ab697c
Heo, J., Hossain, F., & Kim, I. (2020). Challenges in design and fabrication of flexible/stretchable carbon- and textile-based wearable sensors for health monitoring: a critical review. Sensors, 20(14), 3927. https://doi.org/10.3390/s20143927
Jin, C. and Bai, Z. (2022). Mxene-based textile sensors for wearable applications. Acs Sensors, 7(4), 929-950. https://doi.org/10.1021/acssensors.2c00097
Koo, J., Jeong, S., Shim, H., Son, D., Kim, J., Kim, D., … & Kim, D. (2017). Wearable electrocardiogram monitor using carbon nanotube electronics and color-tunable organic light-emitting diodes. Acs Nano, 11(10), 10032-10041. https://doi.org/10.1021/acsnano.7b04292
Lee, J., Park, S., Park, K., & Kim, H. (2019). Flexible and waterproof resistive random‐access memory based on nitrocellulose for skin‐attachable wearable devices. Advanced Functional Materials, 30(1). https://doi.org/10.1002/adfm.201907437
Lyu, Q., Gong, S., Yin, J., Dyson, J., & Cheng, W. (2021). Soft wearable healthcare materials and devices. Advanced Healthcare Materials, 10(17). https://doi.org/10.1002/adhm.202100577
Padash, M., Enz, C., & Carrara, S. (2020). Microfluidics by additive manufacturing for wearable biosensors: a review. Sensors, 20(15), 4236. https://doi.org/10.3390/s20154236
Soler-Crespo, R., Gao, W., Mao, L., Nguyen, H., Roenbeck, M., Paci, J., … & Espinosa, H. (2018). The role of water in mediating interfacial adhesion and shear strength in graphene oxide. Acs Nano, 12(6), 6089-6099. https://doi.org/10.1021/acsnano.8b02373
Wang, H., Li, S., Lü, H., Zhu, M., Liang, H., Wu, X., … & Zhang, M. (2023). Carbon‐based flexible devices for comprehensive health monitoring. Small Methods, 7(2). https://doi.org/10.1002/smtd.202201340
Xu, Y., Fei, Q., Page, M., Zhao, G., Ling, Y., Stoll, S., … & Yan, Z. (2021). Paper-based wearable electronics. Iscience, 24(7), 102736. https://doi.org/10.1016/j.isci.2021.102736
Yang, W., Yang, J., Byun, J., Moissinac, F., Xu, J., Haigh, S., … & Barg, S. (2019). 3d printing of freestanding mxene architectures for current‐collector‐free supercapacitors. Advanced Materials, 31(37). https://doi.org/10.1002/adma.201902725